Single-Photon Emission Computed Tomography
This chapter provides an in-depth overview of SPECT, including instrumentation, quality assurance, as well as compensation methods for image reconstruction. Two overall methods for data acquisition are used in SPECT instrumentation: use of scintillation camera(s) rotating around the subject or multiple detectors arranged in specific geometric patterns around the subject. The latter constitutes dedicated SPECT systems and is mainly used in cardiac applications. We discuss both.
Data Acquisition With a Scintillation Camera
This common approach to SPECT imaging is a natural extension of planar imaging. The scintillation camera is mounted on a rotating gantry, and the acquired data are combined and reconstructed using a software program to generate the transverse cross sections. To reduce the acquisition time further, SPECT systems with two or three scintillation camera heads are preferred. A commercial dual-headed scintillation camera is shown in Figure 14.1.
In this setup (Fig. 14.2), the scintillation camera head (or heads) rotate in a circle around the patient, making a number of stops to acquire data from multiple directions (e.g., ˜60 in routine cardiac imaging). This is a step-and-shoot data acquisition. No data are gathered during movement from one position to the next. To avoid this dead time and increase sensitivity further, acquisition protocols have been developed where data are either gathered continuously (without a stop) or in
a combination of the two (continuous + step-and-shoot), though this can lower the resolution due to camera movement. The orbit of detector heads does not have to be circular. Elliptical or body contour orbits, because of their improved sensitivity and resolution characteristics, are now the standard features.
a combination of the two (continuous + step-and-shoot), though this can lower the resolution due to camera movement. The orbit of detector heads does not have to be circular. Elliptical or body contour orbits, because of their improved sensitivity and resolution characteristics, are now the standard features.
A scintillation camera acquires data simultaneously from a large area of an organ as opposed to a thin section. The data acquired are planar projections of a volume rather than line projections of one (or few) cross sections. Therefore, the data can be easily formatted to produce different cross sections (axial, coronal, sagittal, or oblique) of an organ from a single data acquisition.
Collimators. For optimal performance of SPECT with a scintillation camera, choice of a collimator is very important. For routine use, a general purpose parallel-hole collimator is preferred. A high-resolution parallel-hole collimator may be used in special situations. Special collimators designed for SPECT are also increasingly becoming available. As stated in Chapter 13, iterative
reconstruction algorithms allow for the use of coarser (wider radius and shorter length holes) resolution collimators for matched resolution, but increased sensitivity.
reconstruction algorithms allow for the use of coarser (wider radius and shorter length holes) resolution collimators for matched resolution, but increased sensitivity.
Sources of Error and Needed Quality Control. For an accurate reproduction of a radioactive cross section, the following sources of error are unique for scintillation camera-based SPECT, and require appropriate quality control:
Center of rotation (COR): It is necessary to have proper alignment of the mechanical COR with the COR as defined for reconstruction of projection data. In planar imaging, a shift by one or two pixels in the x or y direction is hardly noticeable, but in SPECT, such a misalignment produces recognizable artifacts (e.g., a point source is reconstructed as a ring). Also, in multiheaded systems, additional alignment in the axial directions between the heads is required. Proper alignment can be achieved by placing a point source at the COR, imaging it from two opposite directions, and determining the pixel location of the source in the two images. If these are identical, then there is no need for adjustment, and the electronic center is properly aligned with the COR. However, if the pixel location of the source is not identical, appropriate adjustments need to be made. This kind of quality assurance is typically performed once every month.
Uniformity: The requirement for uniformity of response of a scintillation camera is more stringent in SPECT than in planar imaging because errors propagate rapidly in reconstruction of the image. Although in planar imaging, uniformity in the range of ˜5% is acceptable, whereas in SPECT it is desirable to be less than 2% to 3%. As explained earlier (p. 147), newer scintillation cameras are capable of such a performance, and flood-field uniformity is measured on a daily basis.
Invariance of uniformity at different angles: Because images are acquired at a large number of angles, the uniformity of a scintillation camera should be maintained at each angle. The uniformity response can change for different angles because the gain for a photomultiplier tube (PMT) can be affected when it is oriented at different angles in the earth’s magnetic field. This effect is in practice minimized by shielding of PMTs with mu-metal (a nickel-iron alloy).
Detector head alignment with the axis of rotation is also another requirement for appropriate image quality as ensured by the manufacturer.
Overall, clinical success of SPECT depends on a stringent quality control program. In addition to this, by acquisition of a sufficient number of counts in each image, use of properly designed collimators, and use of appropriate compensation methods (next), good-quality reconstructed SPECT images are realized routinely in a Clinical Nuclear Medicine Department, as shown in Figure 14.3 for a cardiac study in multiple-gated acquisition scan.
Corrections for Accurate Image Reconstruction
There are three main corrections that can be applied in SPECT reconstruction, namely attenuation correction (AC), scatter correction (SC), and resolution recovery (RR), which can result in improved images, as shown in Figure 14.4. We describe these next.
Attenuation Correction. Image reconstruction in both SPECT and PET is challenged because the γ-rays originating from different pixels pass through different thicknesses of tissues and are therefore attenuated differently. However, there is an additional layer of complication for SPECT. In PET imaging as discussed in Chapter 15, for a given column of pixels, attenuation is independent of the point of origin. This is because, in PET a column (or the so-called line of response) is defined by two detectors that receive the anti-parallel γ-rays (Chapter 15). As a result, the total probability of attenuation only depends on the separation between the two detectors. In SPECT, however, the attenuation is depth-dependent and is different for different pixels in a column.
A number of methods have been used to tackle attenuation in SPECT. These can be classified as analytical or empirical. Analytical methods make simplifying assumptions about attenuation (e.g.,
uniformity of attenuating medium) and are incorporated pre-reconstruction (Sorenson method) or post-reconstruction (Chang method), the latter being more accurate and widely available commercially. These methods, however, fail significantly when the attenuating medium is highly inhomogeneous, such as in the thorax. With empirical methods, the actual attenuation map is obtained by taking multiple transmission scans from multiple projections (e.g., a rotating transmission rod source; similar in principle to a CT scan). This framework produces the best results but requires additional setup and scan time, as well as exposure to the patient, and has not been widely used.
uniformity of attenuating medium) and are incorporated pre-reconstruction (Sorenson method) or post-reconstruction (Chang method), the latter being more accurate and widely available commercially. These methods, however, fail significantly when the attenuating medium is highly inhomogeneous, such as in the thorax. With empirical methods, the actual attenuation map is obtained by taking multiple transmission scans from multiple projections (e.g., a rotating transmission rod source; similar in principle to a CT scan). This framework produces the best results but requires additional setup and scan time, as well as exposure to the patient, and has not been widely used.
![]() Figure 14.3. Cardiac SPECT images. Stress (A) versus rest cardiac (B) images are obtained using 99mTc-Sestamibi (Chapter 5). The stress and rest images are sliced and depicted along cardiac axes, namely the short axis (C, D), the vertical long-axis (E, F), and the horizontal long-axis (G, H). In each of these sets of slices, the top row is for stress, whereas bottom is for rest. |
![]() Figure 14.4. The impact of different image reconstruction and correction methods (AC, SC, and RR) in SPECT imaging. The reconstructions shown involve no post-smoothing. |
However, with the advent of integrated SPECT/CT units (following the very successful adoption of integrated PET/CT; see Chapter 15), the landscape is changing significantly. An example
of a SPECT/CT system is shown in Figure 14.5. In a SPECT/CT system, attenuation maps of the cross-sections are estimated from CT imaging itself. Extrapolation methods are used to transform the CT image, which represents how x-rays (low keV) attenuate in the body, to an attenuation map indicating how γ-rays (high keV) attenuate in the body. Subsequently, iterative reconstruction algorithms are very well suited to incorporate, within their system matrix (Chapter 13), the depth-dependent attenuation factors as determined from these extrapolated attenuation maps. This results in images of improved quality and quantitative accuracy. In the absence of AC, as shown in Figure 14.6 for no correction (N), the image of a uniform source commonly depicts a dip (marked decrease) as one moves toward the center of the object (attenuation artifact). After correction for attenuation, one might be surprised to find an opposite, upward uptake in the center (though not to the same extent). This is also illustrated in Figure 14.6. This is because correcting for attenuation reveals a relatively hidden effect that now needs to be addressed, which brings us to the next point.
of a SPECT/CT system is shown in Figure 14.5. In a SPECT/CT system, attenuation maps of the cross-sections are estimated from CT imaging itself. Extrapolation methods are used to transform the CT image, which represents how x-rays (low keV) attenuate in the body, to an attenuation map indicating how γ-rays (high keV) attenuate in the body. Subsequently, iterative reconstruction algorithms are very well suited to incorporate, within their system matrix (Chapter 13), the depth-dependent attenuation factors as determined from these extrapolated attenuation maps. This results in images of improved quality and quantitative accuracy. In the absence of AC, as shown in Figure 14.6 for no correction (N), the image of a uniform source commonly depicts a dip (marked decrease) as one moves toward the center of the object (attenuation artifact). After correction for attenuation, one might be surprised to find an opposite, upward uptake in the center (though not to the same extent). This is also illustrated in Figure 14.6. This is because correcting for attenuation reveals a relatively hidden effect that now needs to be addressed, which brings us to the next point.
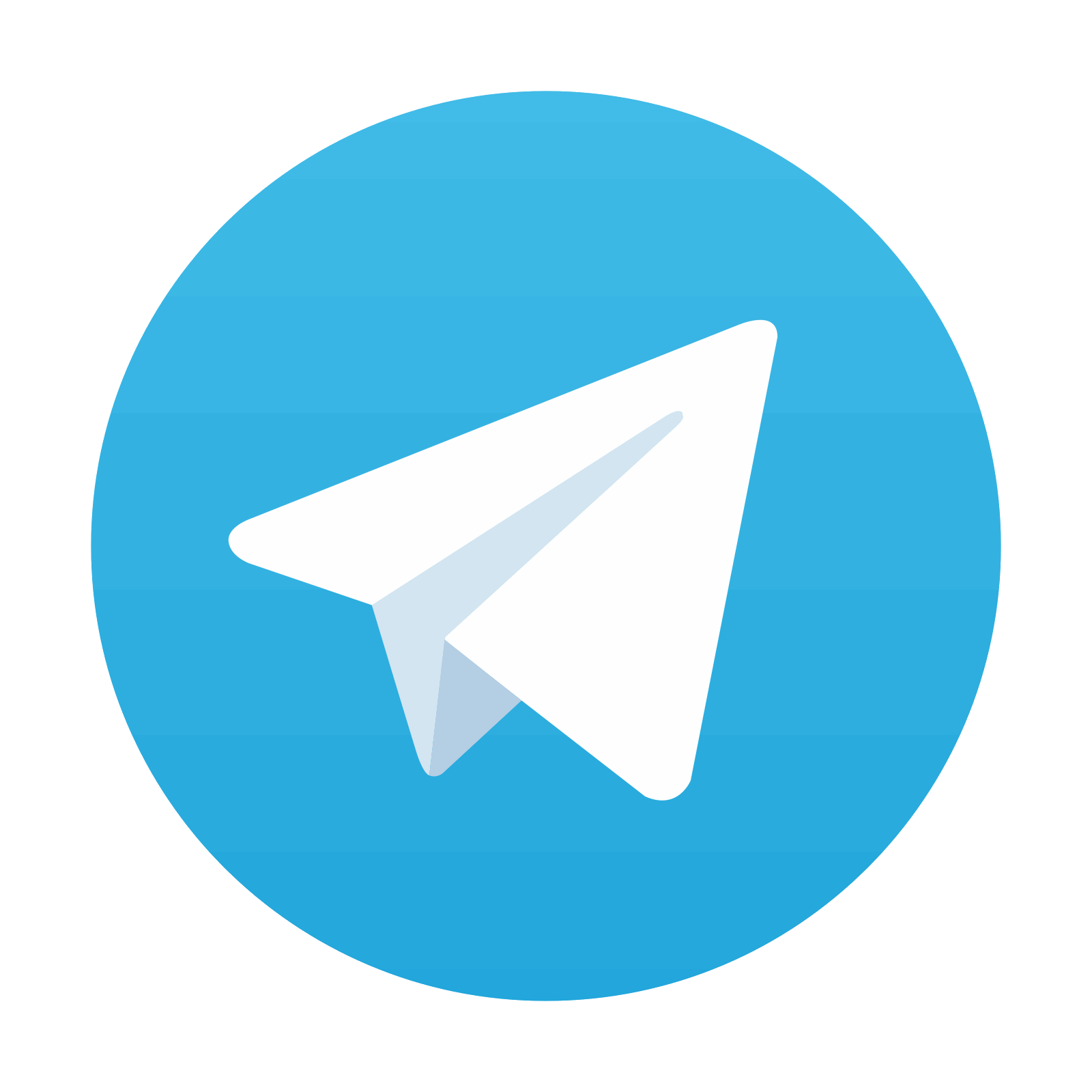
Stay updated, free articles. Join our Telegram channel

Full access? Get Clinical Tree
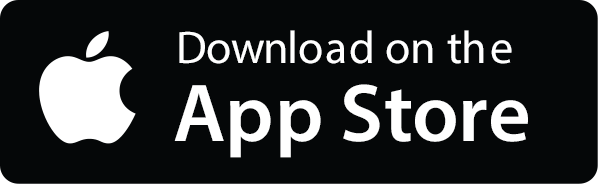
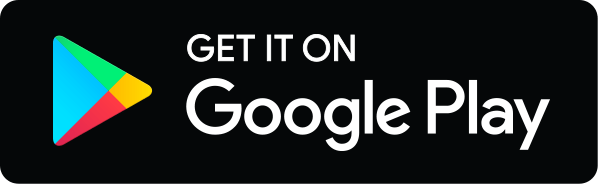