OBJECTIVES
After reading this chapter, youshould be able to:
Describe how the tonicity (osmolality) of the extracellular fluid is maintained byalterations in water intake and vasopressin secretion.
Discuss the effects of vasopressin, the receptors on which it acts, and how its secretion is regulated.
Describe how the volume of the extracellular fluid is maintained by alterations in renin and aldosterone secretion.
Outline the cascade of reactions that lead to the formation of angiotensin II andits metabolites in the circulation.
List the functions of angiotensin II and the receptors on which it acts to carry out these functions.
Describe the structure and functions of atrial natriuretic peptide (ANP), B-type natriuretic peptide (BNP), and C-type natriuretic peptide (CNP) and the receptors on which they act.
Describe the site and mechanism of action of erythropoietin, and the feedback regulation of its secretion.
INTRODUCTION
This chapter is a review of the major homeostatic mechanisms that operate, primarily through the kidneys and the lungs, to maintain the tonicity, the volume, and the specific ionic composition of the extracellular fluid (ECF). The interstitial portion of this fluid is the fluid environment of the cells, and life depends on the constancy of this “internal sea” (see Chapter 1).
DEFENSE OF TONICITY
The defense of the tonicity of the ECF is primarily the function of the vasopressin-secreting and thirst mechanisms. The total body osmolality is directly proportional to the total body sodium plus the total body potassium divided by the total body water, so that changes in the osmolality of the body fluids occur when a mismatch exists between the amount of these electrolytes and the amount of water ingested or lost from the body. When the effective osmotic pressure of the plasma rises, vasopressin secretion is increased and the thirst mechanism is stimulated; water is retained in the body, diluting the hypertonic plasma; and water intake is increased (Figure 38–1). Conversely, when the plasma becomes hypotonic, vasopressin secretion is decreased and “solute-free water” (water in excess of solute) is excreted. In this way, the tonicity of the body fluids is maintained within a narrow normal range. In health, plasma osmolality ranges from 280 mOsm/kg of H2O to 295 mOsm/kg of H2O, with vasopressin secretion maximally inhibited at 285 mOsm/kg and stimulated at higher values (Figure 38–2).
There are at least three kinds of vasopressin receptors: V1A, V1B, and V2. All are G-protein–coupled. The V1A and V1B receptors act through phosphatidylinositol hydrolysis to increase the intracellular Ca2+ concentration. The V2 receptors act through Gs to increase cyclic adenosine 3’,5’-monophosphate (cAMP) levels.
Because one of its principal physiologic effects is the retention of water by the kidney, vasopressin is often called the antidiuretic hormone (ADH). It increases the permeability of the collecting ducts of the kidney, so that water enters the hypertonic interstitium of the renal pyramids. The urine becomes concentrated, and its volume decreases. The overall effect is therefore retention of water in excess of solute; consequently, the effective osmotic pressure of the body fluids is decreased. In the absence of vasopressin, the urine is hypotonic to plasma, urine volume is increased, and there is a net water loss. Consequently, the osmolality of the body fluid rises.
The mechanism by which vasopressin exerts its antidiuretic effect is activated by V2 receptors and involves the insertion of aquaporin 2 into the apical (luminal) membranes of the principal cells of the collecting ducts. Movement of water across membranes by simple diffusion is now known to be augmented by movement through these water channels. These channels are stored in endosomes inside the cells, and vasopressin causes their rapid translocation to the luminal membranes.
V1A receptors mediate the vasoconstrictor effect of vasopressin, and vasopressin is a potent stimulator of vascular smooth muscle in vitro. However, relatively large amounts of vasopressin are needed to raise blood pressure in vivo, because vasopressin also acts on the brain to decrease in cardiac output. The site of this action is the area postrema, one of the circumventricular organs (see Chapter 33). Hemorrhage is a potent stimulus for vasopressin secretion, and the blood pressure fall after hemorrhage is more marked in animals that have been treated with synthetic peptides that block the pressor action of vasopressin. Consequently, it appears that vasopressin does play a role in blood pressure homeostasis.
V1A receptors are also found in the liver and the brain. Vasopressin causes glycogenolysis in the liver, and, as noted above, it is a neurotransmitter in the brain and spinal cord.
The V1B receptors (also called V3 receptors) appear to be unique to the anterior pituitary, where they mediate increased secretion of adrenocorticotropic hormone (ACTH) from the corticotropes.
Circulating vasopressin is rapidly inactivated, principally in the liver and kidneys. It has a biologic half-life of approximately 18 min in humans.
Vasopressin is stored in the posterior pituitary and released into the bloodstream in response to impulses in the nerve fibers that contain the hormone. The factors affecting its secretion are summarized in Table 38–1. When the effective osmotic pressure of the plasma is increased above 285mOsm/kg, the rate of discharge of neurons containing vasopressin increases and vasopressin secretion occurs (Figure 38–2). At 285 mOsm/kg, plasma vasopressin is at or near the limits of detection by available assays, but its levels probably decrease when plasma osmolality is below this level. Vasopressin secretion is regulated by osmoreceptors located in the anterior hypothalamus. They are outside the blood-brain barrier and appear to be located in the circumventricular organs, primarily the organum vasculosum of the lamina terminalis (OVLT) (see Chapter 33). The osmotic threshold for thirst (Figure 38–1) is the same as or slightly greater than the threshold for increased vasopressin secretion (Figure 38–2), and it is still uncertain whether the same osmoreceptors mediate both effects.
FIGURE 38–2
Relation between plasma osmolality and plasma vasopressin in healthy adult humans during infusion of hypertonic saline. LD, limit of detection. (Reproduced with permission from Thompson CJ, et al: The osmotic thresholds for thirst and vasopressin are similar in healthy humans. Clin Sci [Colch] 1986; Dec; 71(6):651–656.)
Vasopressin Secretion Increased | Vasopressin Secretion Decreased |
---|---|
Increased effective osmotic pressure of plasma Decreased ECF volume Pain, emotion, “stress,” exercise Nausea and vomiting Standing Clofibrate, carbamazepine Angiotensin II | Decreased effective osmotic pressure of plasma Increased ECF volume Alcohol |
Vasopressin secretion is thus controlled by a delicate feedback mechanism that operates continuously to defend the osmolality of the plasma. Significant changes in secretion occur when osmolality is changed as little as 1%. In this way, the osmolality of the plasma in normal individuals is maintained very close to 285 mOsm/L.
ECF volume also affects vasopressin secretion. Vasopressin secretion is increased when ECF volume is low and decreased when ECF volume is high (Table 38–1). There is an inverse relationship between the rate of vasopressin secretion and the rate of discharge in afferents from stretch receptors in the low- and high-pressure portions of the vascular system. The low-pressure receptors are those in the great veins, right and left atria, and pulmonary vessels; the high-pressure receptors are those in the carotid sinuses and aortic arch (see Chapter 32). The exponential increases in plasma vasopressin produced by decreases in blood pressure are documented in Figure 38–3. However, the low-pressure receptors monitor the fullness of the vascular system, and moderate decreases in blood volume that reduce central venous pressure without lowering arterial pressure can also increase plasma vasopressin.
FIGURE 38–3
Relation of mean arterial blood pressure to plasma vasopressin in healthy adult humans in whom a progressive decline in blood pressure was induced by infusion of graded doses of the ganglionic blocking drug trimethaphan. The relation is exponential rather than linear. (Data from Baylis PH: Osmoregulation and control of vasopressin secretion in healthy humans. Am J Physiol 1987;253:R671.)
Thus, the low-pressure receptors are the primary mediators of volume effects on vasopressin secretion. Impulses pass from them via the vagi to the nucleus of the tractus solitarius (NTS). An inhibitory pathway projects from the NTS to the caudal ventrolateral medulla (CVLM), and there is a direct excitatory pathway from the CVLM to the hypothalamus. Angiotensin II reinforces the response to hypovolemia and hypotension by acting on the circumventricular organs to increase vasopressin secretion (see Chapter 33).
Hypovolemia and hypotension produced by conditions such as hemorrhage release large amounts of vasopressin, and in the presence of hypovolemia, the osmotic response curve is shifted to the left (Figure 38–4). Its slope is also increased. The result is water retention and reduced plasma osmolality. This includes hyponatremia, since Na+ is the most abundant osmotically active component of the plasma.
FIGURE 38–4
Effect of hypovolemia and hypervolemia on the relation between plasma vasopressin (pAVP) and plasma osmolality. Seven blood samples were drawn at various times from 10 normal men when hypovolemia was induced by water deprivation (green circles, dashed line) and again when hypervolemia was induced by infusion of hypertonic saline (red circles, solid line). Linear regression analysis defined the relationship pAVP = 0.52 (plasma osmolality – 283.5) for water deprivation and pAVP = 0.38 (plasma osmolality – 285.6) for hypertonic saline. LD, limit of detection. Note the steeper curve as well as the shift of the intercept to the left during hypovolemia. (Used with permission of CJ Thompson.)
A variety of stimuli in addition to osmotic pressure changes and ECF volume aberrations increase vasopressin secretion. These include pain, nausea, surgical stress, and some emotions (Table 38–1). Nausea is associated with particularly large increases in vasopressin secretion. Alcohol decreases vasopressin secretion.
In various clinical conditions, volume and other nonosmotic stimuli bias the osmotic control of vasopressin secretion. For example, patients who have had surgery may have elevated levels of plasma vasopressin because of pain and hypovolemia, and this may cause low plasma osmolality and dilutional hyponatremia (see Clinical Box 38–1).
Diabetes insipidus is the syndrome that results when there is a vasopressin deficiency (central diabetes insipidus) or when the kidneys fail to respond to the hormone (nephrogenic diabetes insipidus).
Causes of vasopressin deficiency include disease processes in the supraoptic and paraventricular nuclei, the hypothalamohypophysial tract, or the posterior pituitary gland. It has been estimated that 30% of clinical cases are due to neoplastic lesions of the hypothalamus, either primary or metastatic; 30% are posttraumatic; 30% are idiopathic; and the remainder are due to vascular lesions, infections, systemic diseases such as sarcoidosis that affect the hypothalamus, or mutations in the gene for prepropressophysin. Disease that develops after surgical removal of the posterior lobe of the pituitary may be temporary if the distal ends of the supraoptic and paraventricular fibers are only damaged, because the fibers recover, make new vascular connections, and begin to secrete vasopressin again.
The symptoms of diabetes insipidus are passage of large amounts of dilute urine (polyuria) and the drinking of large amounts of fluid (polydipsia), provided the thirst mechanism is intact. It is the polydipsia that keeps these patients healthy. If their sense of thirst is depressed for any reason and their intake of dilute fluid decreases, dehydration that can be fatal develops.
Another cause of diabetes insipidus is inability of the kidneys to respond to vasopressin (nephrogenic diabetes insipidus). Two forms of this disease have been described. In one form, the gene for the V2 receptor is mutated, making the receptor unresponsive. The V2 receptor gene is on the X chromosome, thus this condition is X-linked and inheritance is sex-linked recessive. In the other form of the condition, mutations occur in the autosomal gene for aquaporin-2 and produce nonfunctional versions of this water channel, many of which do not reach the apical membrane of the collecting duct but are trapped in intracellular locations.
CLINICAL BOX 38–1 Syndrome of Inappropriate Antidiurectic Hormone
The syndrome of “inappropriate” hypersecretion of antidiuretic hormone (SIADH) occurs when vasopressin is inappropriately high relative to serum osmolality. Vasopressin is responsible not only for dilutional hyponatremia (serum sodium < 135 mmol/L) but also for loss of salt in the urine when water retention is sufficient to expand the ECF volume, reducing aldosterone secretion (see Chapter 20). This occurs in patients with cerebral disease (“cerebral salt wasting”) and pulmonary disease (“pulmonary salt wasting”). Hypersecretion of vasopressin in patients with pulmonary diseases such as lung cancer may be due in part to the interruption of inhibitory impulses in vagal afferents from the stretch receptors in the atria and great veins.
A significant number of lung tumors and some other cancers secrete vasopressin. There is a process called “vasopressin escape” that counteracts the water-retaining action of vasopressin to limit the degree of hyponatremia in SIADH. Studies in rats have demonstrated that prolonged exposure to elevated levels of vasopressin can lead eventually to down-regulation of the production of aquaporin-2. This permits urine flow suddenly to increase and plasma osmolality to fall despite exposure of the collecting ducts to elevated levels of the hormone; that is, the individual escapes from the renal effects of vasopressin.
THERAPEUTIC HIGHLIGHTSPatients with inappropriate hypersecretion of vasopressin have been successfully treated with demeclocycline, an antibiotic that reduces the renal response to vasopressin.
Synthetic peptides that have selective actions and are more active than naturally occurring vasopressin have been produced by altering the amino acid residues. For example, 1-deamino-8-D-arginine vasopressin (desmopressin; dDAVP) has very high antidiuretic activity with little pressor activity, making it valuable in the treatment of vasopressin deficiency.
DEFENSE OF VOLUME
The volume of the ECF is determined primarily by the total amount of osmotically active solute in the ECF. The composition of the ECF is discussed in Chapter 1. Because Na+ and Cl− are by far the most abundant osmotically active solutes in ECF, and because changes in Cl− are to a great extent secondary to changes in Na+, the amount of Na+ in the ECF is the most important determinant of ECF volume. Therefore, the mechanisms that control Na+
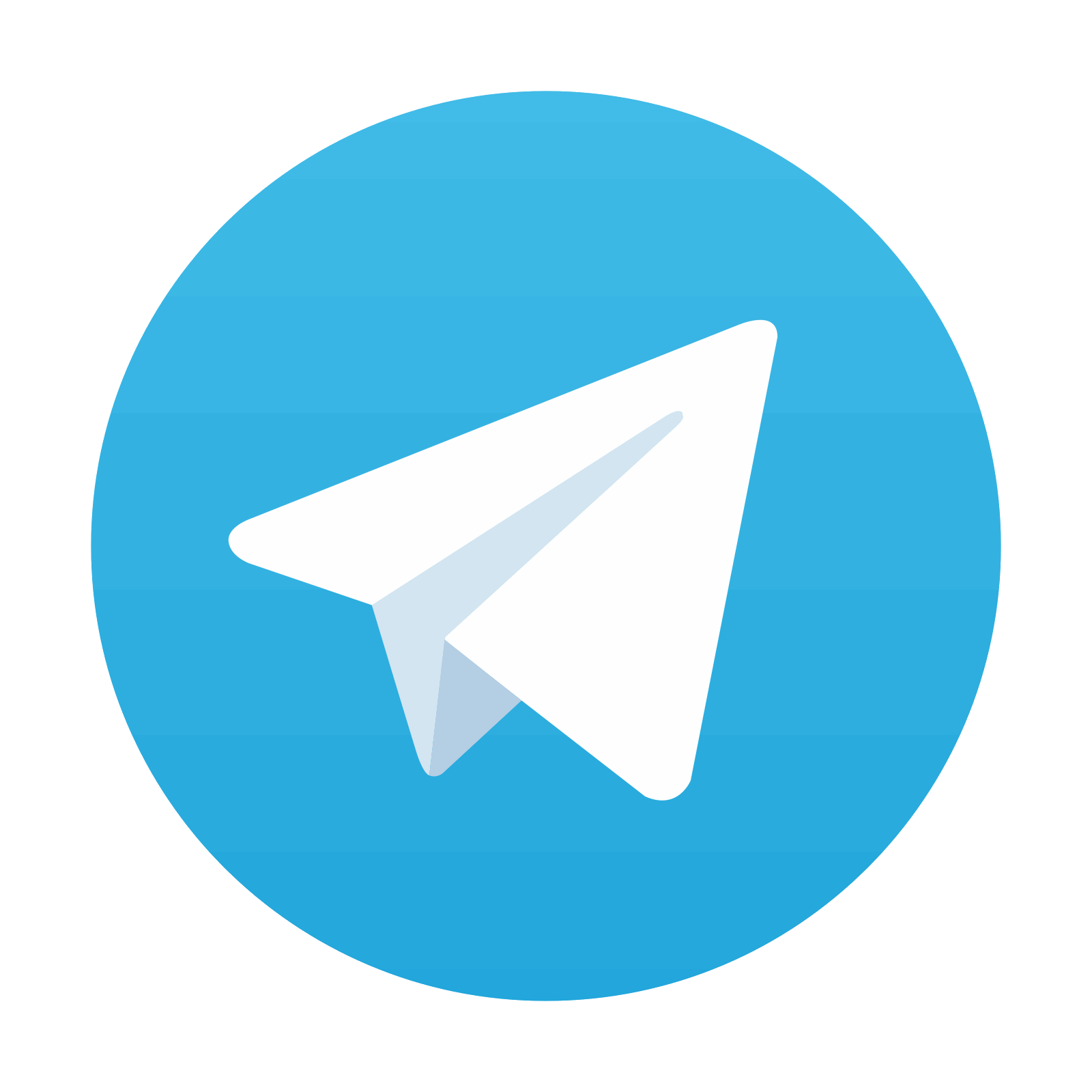
Stay updated, free articles. Join our Telegram channel

Full access? Get Clinical Tree
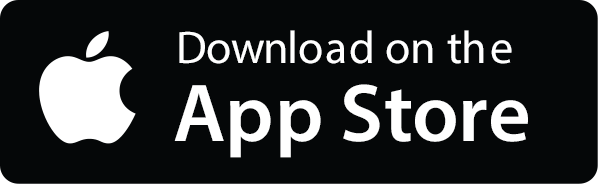
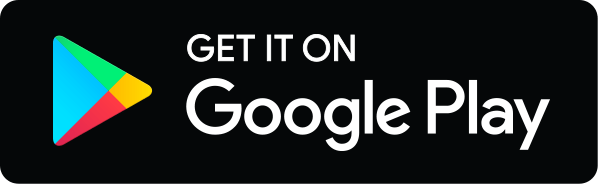
