Whilst the practice of pharmacology is to ultimately reach an end goal of understanding pharmacodynamics (what the drug does to the body), scientists and clinicians also need to understand what the body does to the drug (pharmacokinetics). This is a vitally important aspect of pharmacology both in drug development and also in the practice of medicine, where individuals, populations and ethnic groups will vary considerably in their response to drugs, and the dose and frequency will need adjusting accordingly to control the amount of drug within the ‘therapeutic window’. Pharmacokinetics therefore provides an understanding of the fate of the dose of drug administered, and the time course and levels of unbound drug in the body. This is influenced by factors determining drug disposition that is divided into four stages and is recognized by the acronym ‘ADME’:
- •
Absorption from the site of administration
- •
Distribution within the body
- •
Metabolism
- •
Excretion
Absorption and Distribution of Drugs
It is important to know how drug concentrations in tissues change with time, i.e. to understand the pharmacokinetic aspects of drug action. The time course of drug action usually follows that of the concentration at the target site. Important exceptions are drugs that bind irreversibly (e.g. organophosphorus anticholinesterases), where the effect can outlive the concentration. Following absorption, drugs are carried to their targets in the body and their sites of elimination by the blood circulation. Well-perfused tissues, such as the lungs and kidney, can equilibrate with the plasma concentration quickly, whereas poorly perfused tissues, such as fat, will only take up and release drugs slowly. Once a drug arrives at a tissue, it must pass out of the blood capillaries and possibly cross cell membrane barriers, either to reach its target or to pass into the urine or bile. Accordingly, a drug’s ability to cross cell membranes is of major concern in pharmacology.
Membrane permeation by drugs
The plasma membrane of cells constitutes a hydrophobic lipid barrier and drug permeation can occur by:
- •
direct diffusion through the lipid,
- •
carrier-mediated transport,
- •
diffusion through aqueous pores, or
- •
pinocytosis.
The first two mechanisms are most important; aqueous pores are too small to allow the passage of most drugs (which typically have molecular weights in the range 200–1000) and pinocytosis is thought to be important for only a few large molecules (e.g. insulin penetration of the blood–brain barrier).
Diffusion through membrane lipids
Diffusion of a drug depends on its concentration gradient and its diffusion coefficient. The concentration gradient established within the cell membrane depends on the drug’s lipid/water partition coefficient. This is conveniently estimated by the drug’s distribution between water and a simple organic solvent, such as heptane. (There is a good correlation between such partition coefficients and drug absorption.)
Ionization
Most drugs, being weak acids or weak bases, ionize to some extent in aqueous solution. The ionized form is lipophobic , so that ionization impedes passive membrane permeation.
The fractional ionization can be determined from the Henderson–Hasselbalch equation:
foraweakacid:log10cicu=pH-pKa
foraweakbase:log10cicu=pKa-pH
Ionization thus depends on the pH of the aqueous environment and the drug’s acid dissociation constant (a strong acid has a low p K a and a strong base has a high p K a ; see Fig. 5.1 ).

Ion trapping
Where a lipid membrane separates solutions of different pH, the difference in ionization on the two sides can lead to an uneven distribution. The ionized molecules do not readily cross the membrane and there is an effective trapping of these molecules on the side promoting ionization. For example, a weak base such as morphine, even when given intravenously, will achieve a high concentration in the acidic gastric lumen ( Fig. 5.2 ).

Active transport
Carriers are important for membrane transport of essential nutrients that have low lipid solubility. Carriers can be classified into solute carrier (SLC) transporters (aid the passive transport of solutes down their electrochemical gradient) and ATP-binding cassette (ABC) transporters (pumps that are active and require ATP as an energy source) . SLCs may be further classified as organic cation transporters (OCTs) and organic anion transporters (OATs). Most drugs are exogenous substances of no nutritional value and are not substrates for the carriers involved in nutrient absorption or delivery to cells (exceptions include the anticancer agent 5-fluorouracil, an analogue of uracil, which can use the transporter for pyrimidines in the gut). However, SLCs are important for carrier-mediated drug transport in the blood-brain barrier, gastrointestinal tract, renal tubules, biliary tract, and the placenta.
Active transport systems in the kidney and liver are, by comparison, very important in the elimination of drugs from the body. (Such carriers have probably evolved for the clearance of toxic substances that might have been ingested and whose rapid elimination would have survival value.) Active transport (via the P-glycoprotein transporter) is also an important mechanism for resistance to anticancer drugs.
Features of active drug transport are:
- •
uphill transport (allowing high renal clearances) and
- •
a finite number of transporter molecules leading to:
- –
saturation and
- –
competition between drugs for transport, exacerbated by the low substrate specificity of many carriers.
- –
Drug absorption
Drugs can be administered via the gut (enteral) or by other routes (parenteral), and the route will be chosen based on a number of factors concerning the chemical properties of the drug that will affect its ADME profile, the target organ and safety ( Fig. 5.3 ).

Enteral administration
Oral absorption from the stomach or intestines, after the drug is swallowed, is the most convenient and acceptable route. An important consideration is that oral absorption will deliver the drug directly to the liver, the major site of drug metabolism, via the portal circulation; this may result in substantial first-pass elimination . Drugs absorbed from the mouth ( sublingual or buccal) and the lower rectum do not enter the hepatic portal vein and so avoid first-pass metabolism. Sublingual glyceryl trinitrate is rapidly absorbed to provide quick relief of anginal pain (it is ineffective if swallowed). Rectal administration is suitable for some irritants and when vomiting prevents oral medication.
Factors controlling oral absorption
Lipid solubility and ionization
This is discussed above. Note that the intestine with its villi and microvilli is the main absorptive area, so that weak acids, which will be less ionized in the stomach, will, nevertheless, be mostly absorbed in the more alkaline intestine. Rapid passage of drug from the stomach to intestine is likely to speed up drug absorption.
Drug formulation
Drugs must dissolve to establish a concentration gradient for absorption, the rate and extent of absorption depending on the pharmaceutical formulation. Rapid absorption of a tablet requires its disintegration into small particles that readily dissolve. Note that while a drug is more water soluble in its ionized form, it is the unionized form that is membrane permeable. Sustained release formulations release the drug slowly to prolong drug action and reduce the frequency of administration.
Gastrointestinal motility
Stasis can slow oral absorption while diarrhoea, predictably, may allow insufficient time for complete absorption.
Interactions with other substances in the gut
Food will often slow absorption by simply reducing the drug’s concentration. More specific interactions can also occur; for example tetracyclines will interact with Ca 2+ in food to form an incompletely absorbed, insoluble complex.
Bioavailability
Bioavailability is a term applied particularly to oral preparations and expresses the extent to which a dose is absorbed. The most useful definition is the proportion of the administered dose that reaches the systemic circulation . Incomplete release from the dosage form, destruction within the gut, poor absorption and first-pass elimination are important causes of low bioavailability. For drugs with a low therapeutic index, it is important that repeat prescriptions provide medicines of equivalent bioavailability ( bioequivalence ).
Parenteral administration
These routes are useful for:
- •
rapid effects (e.g. in status asthmaticus),
- •
drugs that are poorly absorbed from the gut (e.g. pancuronium, insulin),
- •
irritants (some anticancer agents), and
- •
localization of action (e.g. inhaled bronchodilators, mydriatics).
Intravenous (IV) injection is the most rapid route, IV infusions allowing tight control of drug concentration in the plasma. Intramuscular (IM) and subcutaneous (SC) injection have found particular utility in providing long-term therapy from depot preparations (e.g. contraceptive steroid implants ( Ch. 17 ) or slowly released antipsychotic agents ( Ch. 12 )). Inhalation is used for anaesthetic gases ( Ch. 27 ) and in treatment of bronchial asthma and chronic obstructive pulmonary disease (COPD) with bronchodilators and antiinflammatory steroids ( Chapter 16, Chapter 20 ).
Topical application of drugs to the skin is used mainly for local actions, but systemic absorption of very lipid-soluble substances is possible (e.g. scopolamine patches to prevent motion sickness).
Drug distribution
Most drugs entering the body do not spread rapidly throughout the whole of body water to achieve a uniform concentration within the major compartments (plasma, interstitial fluid, intracellular fluid, transcellular fluid and fat). Large molecules (heparin, insulin) cannot easily enter interstitial and intracellular spaces whereas smaller and lipid-soluble molecules can.
A drug’s penetration into these compartments is indicated by its apparent volume of distribution ( V d ): the volume of fluid that would be required to hold the amount of drug in the body at the measured plasma concentration. It can be estimated by the equation:
Vd=Dose/cp
- •
Heparin: 0.05–0.1
- •
Tubocurarine: 0.2–0.4
- •
Ethanol: 1.0
- •
Propranolol: 2–5
- •
Nortriptyline: >20
The ‘volume of distribution’ is not a real ‘volume’, but a parameter of the amount of drug in the plasma relative to the total amount of drug in the body. V d is determined by the binding of drug to tissue compared to plasma protein. A large V d is suggestive of a drug tightly bound to tissues and little in the blood plasma. Thus in the examples above, heparin (which is a very large, highly charged drug) is held in blood plasma to a far greater extent than propranolol or nortriptyline. Lipid-insoluble drugs are mainly confined to plasma and interstitial fluids, whereas lipid-soluble drugs reach all compartments and may accumulate in fat.
V d is used to determine the loading dose of drugs, that is the initial dose of drug administered to reach steady state more quickly, than if the maintenance dose was used where clearance might delay the level of drug concentration needed to reach a therapeutic level.
Binding to protein and other tissue components
Tetracyclines bind to calcium in bones and teeth (which can produce abnormalities in tooth development in children). Many drugs bind to plasma proteins of which albumin is generally the most important, although α-acid glycoprotein is of greater importance for some basic drugs (e.g. propranolol). For some drugs, more than 95% of drug in plasma may be bound to protein. Binding to plasma proteins is of finite capacity and of low specificity and has several consequences:
- •
The bound drug is usually inactive.
- •
The reduction in free drug concentration may reduce elimination (by reducing glomerular filtration) or, conversely, protein binding may serve to deliver drug to the kidney and liver, and so enhance elimination.
- •
One drug may prevent the binding of another, and so enhance pharmacological activity. (This is of significance only for highly bound drugs, such as warfarin (98% bound), whose displacement by just 2% would double the free concentration. Thus, changes by only a fraction will result in increased activity and thus can lead to serious side effects such as bleeding.)
Accumulation in lipid
Lipid-soluble drugs may concentrate in adipose tissue which is a large non-polar compartment. For example, halothane can concentrate in fat during long operations and its slow release can lead to prolonged CNS depression postoperatively. Certain pesticides such as DDT can also accumulate in fat and this can lead to long-term neurotoxicity.
Penetration of drugs into the brain
The endothelial cells lining brain capillaries are joined to each other by tight junctions to produce an unbroken cell membrane lining, which is the main element of the blood–brain barrier. This prevents passive entry into the brain of lipophobic/ionized molecules. An additional feature is the turnover of cerebrospinal fluid. This is produced by the choroid plexuses, flows through the ventricles and after reaching the outer surface of the brain drains into the blood at the arachnoid villi. Drugs that penetrate slowly will be removed by ‘washout’ in this way and achieve a steady-state concentration much below the plasma concentration.
Improvements in drug delivery
Pharmaceutical scientists have developed technologies to overcome some of the issues described above in order to improve drug delivery and tissue localization. Pro-drugs, biologically erodible nanoparticles, antibody-drug conjugates, packaging in liposomes, and coatable implantable devices are examples of how this may be achieved.
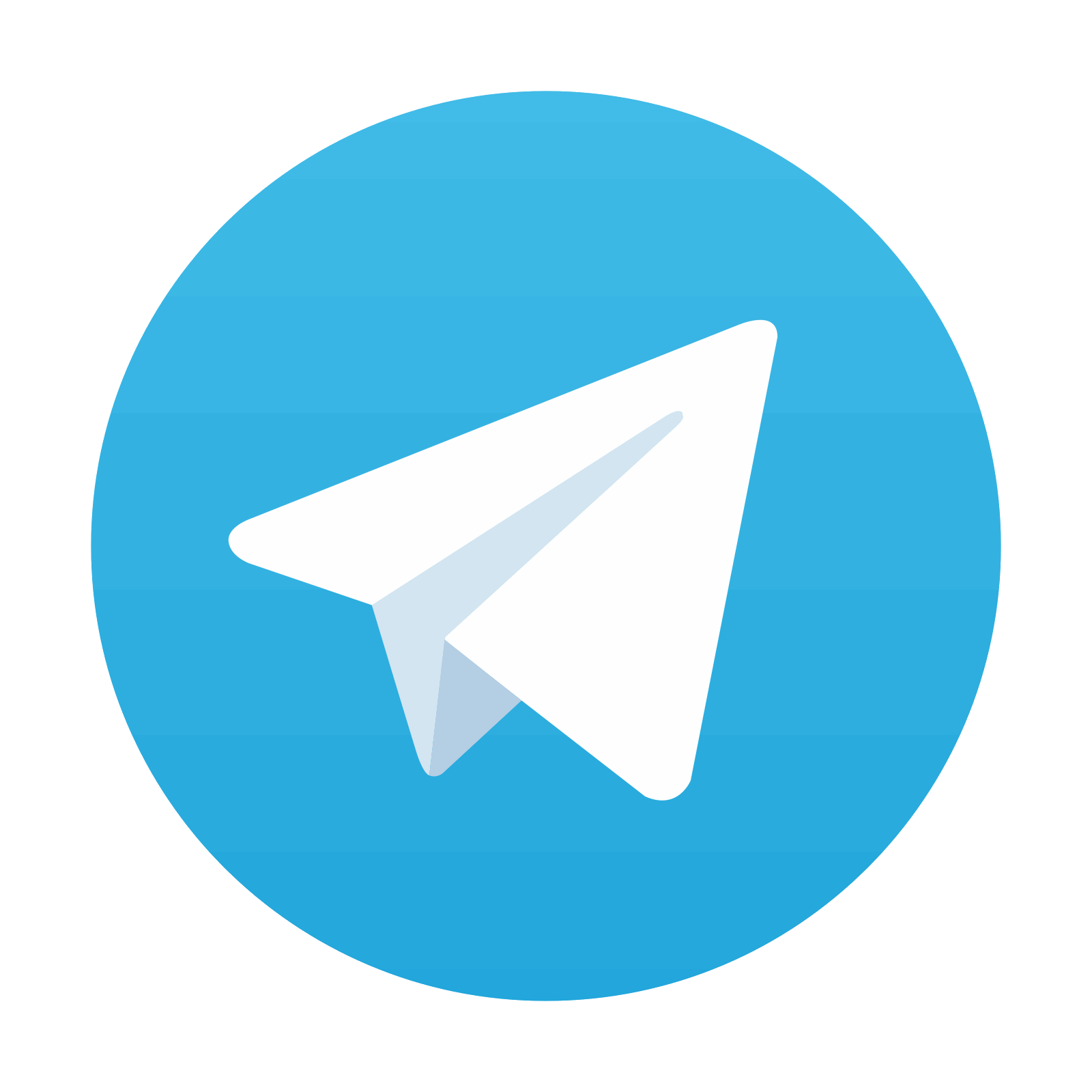
Stay updated, free articles. Join our Telegram channel

Full access? Get Clinical Tree
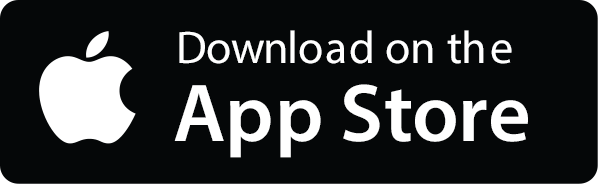
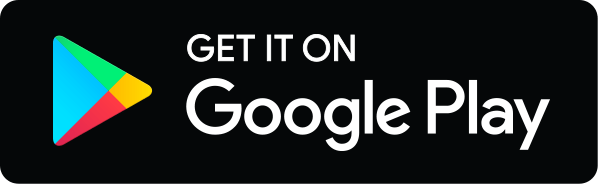