Pharmacogenomics: Introduction
The study of pharmacogenomics addresses the interactions of multiple genes and gene products and their impact on drug therapy, with the goal of developing rational means to optimize drug therapy and ensure maximal efficacy with minimal side effects. In a gross way physicians already use pharmacogenomics when choosing cardiac drugs for patients. For example, hypertensive patients of African background and black race tend to respond better to diuretics and worse to angiotensin-converting enzyme inhibitors and β-blockers. A study investigating congestive heart failure in blacks was terminated prematurely because of an absolute risk reduction in the death rate of 4%. The Food and Drug Administration (FDA) then approved the combination of two well-known drugs, isosorbide and hydralazine, as an adjunct to standard treatment of heart failure in African Americans.
In practice, however, humans and their genome are much more complicated than a simple classification based on race. For example, hypertension in African Americans is higher than that in Caucasian Americans, but the same among African Cubans and Caucasian Cubans. Furthermore, studies of human genetic diversity often sample small numbers of members of a racial or ethnic group, and importantly, membership in a particular ethnic group or race is often defined more by the subject’s ethnic identification than by objective criteria. In addition, genetic studies are not usually designed to assess other important influences on medical phenomena such as environment, social class, poverty, and lifestyle. Using race to guide prescription of medication is a proxy for understanding the underlying genetic, environmental, social, economic, and lifestyle causes of illness.
Genetic Variability & Its Effects on Pharmacokinetics & Pharmacodynamics
The effect of drugs is traditionally divided into pharmacokinetics (how drugs are absorbed, distributed, metabolized, and eliminated) and pharmacodynamics (target or targets underlying the therapeutic effect). In principle, genetic variation can influence either pharmacokinetics or pharmacodynamics, or both. Currently, most clinical application of pharmacogenetics involves pharmacokinetics, but over time more attention will shift to pharmacodynamics. The sequencing of the human genome and intensive research into how genetic variation affects drug response holds the promise of altering the paradigms for medication therapy. However, as will be discussed later, current clinical applications utilizing pharmacogenetics are still rather limited. The coming years should see steady growth in this field that will allow primary care providers and other health professionals to better manage drug therapy.
The most common type of genetic variation is single-nucleotide polymorphism (SNP), a situation in which some individuals have one nucleotide at a given position while other individuals have another nucleotide (eg, cytosine versus adenosine, C/A). If this occurs in the coding region of a gene (ie, within coding exons), this may or may not result in a change in the amino acid sequence that results when DNA is transcribed into RNA and the RNA is then translated into protein. SNPs that cause a change in amino acid sequence are termed nonsynonymous whereas SNPs that do not change amino acid sequence are termed synonymous. Typically, synonymous substitutions are thought of as neutral, as assumption that is generally but not always true (an example of this is seen in the gene responsible for cystic fibrosis). Other less common types of genetic variation include insertions or deletions (sometimes referred to collectively as “indels”), partial or total gene deletion, alteration of mRNA splicing (ie, the process of removing introns from genomic DNA sequences that contain exons and introns), variation in gene promoters, and gene duplication or multiplication.
Introduction to some terms and nomenclature used in pharmacogenetics is helpful. In dealing with pharmacogenetic variation of drug-metabolizing enzymes, particularly the cytochrome P450 (CYP) enzymes, poor metabolizers represent individuals with little or no enzyme activity, either due to lack of expression of the enzyme or mutations that reduce enzymatic activity (eg, a mutation that alters the active catalytic site). Extensive metabolizers are considered the “normal” situation and generally represent individuals with two normal copies of the enzyme gene on each chromosome. Intermediate metabolizers have enzyme activity roughly half that of extensive metabolizers. The most common genetic reason underlying intermediate activity is one copy of the normal gene and one variant copy associated with low activity. Ultrarapid metabolizers have enzyme activity significantly greater than the average population. This is often due to the individual having duplicated or multiplicated copies of a gene (ie, more than the normal two copies of a gene, one on each chromosome). Gene duplication or multiplication is not seen with many genes but can occur with CYP 2D6. For example, an individual may have three or more functional copies of the CYP 2D6 gene instead of the normal two copies.
Genetic variants involving drug-metabolizing enzymes are named in a historical but often confusing system to those new to the field. By definition, the normal allele (individual copy of a gene on a chromosome) is defined as *1 (eg, CYP 2D6*1). In order of historical discovery, variant alleles were designated *2, *3, *4, and so on, in some cases with subtypes (eg, *3A, *3B) added later on. Unfortunately, this nomenclature does not give any clue to the nature of the genetic variation. For instance, *4 and *6 could represent fairly benign genetic variants whereas *5 could signify a variant that results in complete absence of enzyme activity.
Although a number of nongenetic factors influence the effects of medications—including disease, organ function, concomitant medications, herbal therapy, age, and gender—there are now many examples in which interindividual differences in medication response are due to variants in genes encoding drug targets, drug-metabolizing enzymes, and drug transporters. Currently, most well-established applications of pharmacogenetics involve pharmacokinetics.
Several organs can metabolize (biotransform) drug molecules, with the liver being the dominant organ for this purpose in humans and other mammals. The proteins that have been studied in greatest depth are the CYP enzymes, a complicated group of enzymes expressed in liver, intestines, kidneys, lungs, and some other organs. Several CYP enzymes account for the majority of drug metabolism in humans: CYP 3A4/5, CYP 2D6, CYP 2C9, and CYP 2C19. CYP 3A4, in particular, has been shown to play a role in the metabolism of over 50% of the prescribed drugs in the United States.
Genetic variation of CYP 2D6 was one of the first classic examples of pharmacogenetics. In the 1970s, the experimental (and now obsolete) antihypertensive drug debrisoquine was being tested. For the majority of individuals, this drug provided safe control of hypertension. However, some individuals developed prolonged hypotension after receiving the drug, with the hypotension lasting for days in some people. Debrisoquine was later found to be mainly metabolized by CYP 2D6, an enzyme found in the liver that is now known to metabolize about 25% of all drugs currently prescribed in the United States. Of the CYP enzymes, CYP 2D6 is the most highly genetically polymorphic (ie, it shows the greatest range of genetic variation), with reasonably common genetic variants that include total gene deletion (CYP 2D6*5) and gene duplication, with rare individuals documented that have over 10 functional copies of the CYP 2D6 gene. Genetic variation of CYP 2D6, as will be discussed below, has clinical importance for psychiatric, cardiac, and opiate medications. CYP 2D6 poor metabolizers may experience severe adverse effects to standard doses of certain drugs whereas ultrarapid metabolizers may degrade a drug so quickly that therapeutic concentrations are not achieved with standard doses.
The CYP enzymes also underlie a number of clinically important drug-drug or drug-food interactions. For example, ketoconazole is a powerful inhibitor of multiple CYP enzymes, including CYP 3A4. Ketoconazole thus has potentially dangerous interactions with drugs metabolized by CYP 3A4 such as the immunosuppressive drug cyclosporine, if appropriate dose reductions are not made. On the contrary, several compounds markedly increase (induce) the expression of CYP enzymes, including rifampin, phenytoin, carbamazepine, phenobarbital, and the herbal antidepressant St. John’s wort. By increasing the expression of CYP enzymes and other proteins involved in drug metabolism and elimination, inducers such as rifampin can cause increased metabolism not only of other drugs but also of endogenous compounds such as steroid hormones and vitamin D. This is the mechanism underlying unintended pregnancy that can result in women using estrogen-containing oral contraceptives who also receive a CYP inducer such as rifampin. CYP inducers greatly increase the metabolism of the estrogen component of combined oral contraceptives, resulting in therapeutic failure. CYP inducers are also known to cause osteomalacia by accelerating the metabolism and clearance of the active form of vitamin D (1α,25-dihydroxyvitamin D3).
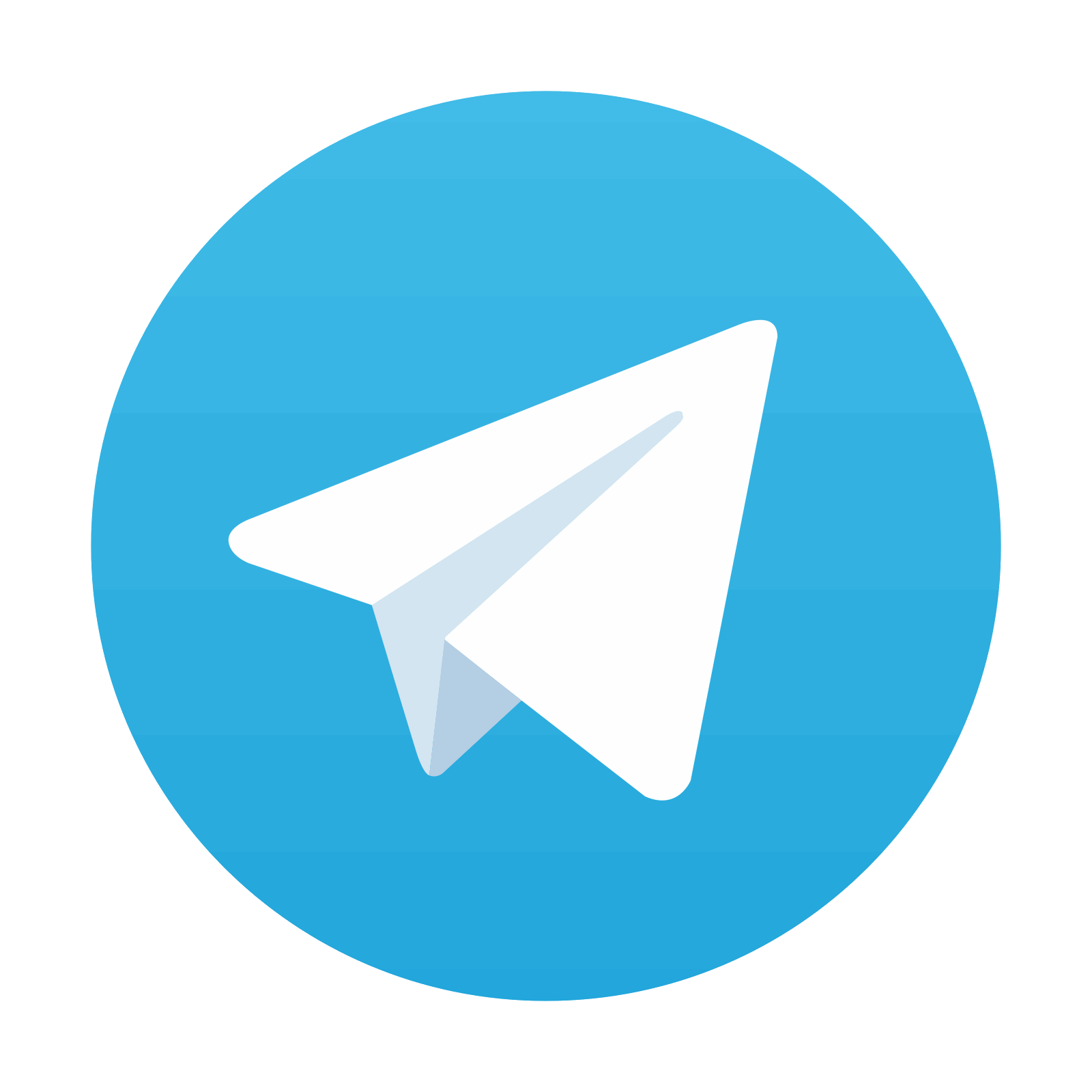
Stay updated, free articles. Join our Telegram channel

Full access? Get Clinical Tree
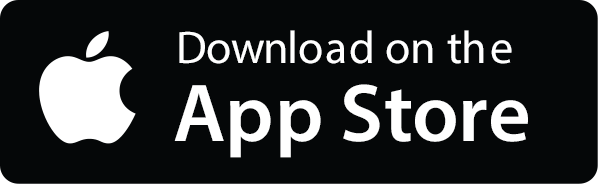
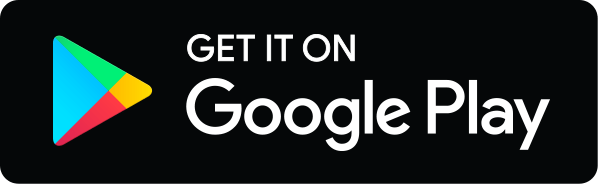