it is bound by transferrin and circulates within the blood. In healthy individuals, the blood contains much more transferrin protein than iron, and the transferrin proteins are approximately 30% saturated with iron. As blood iron levels increase, the excess transferrin proteins buffer the excess iron to help prevent toxicity. Saturation levels of 45% and above are associated with excess body iron stores.
Table 17.1 Important proteins and cells that play a role in iron metabolism | ||||||||||||||||||||||||||||
---|---|---|---|---|---|---|---|---|---|---|---|---|---|---|---|---|---|---|---|---|---|---|---|---|---|---|---|---|
|
basis. A genetic link was subsequently confirmed in 1975 by Simon and colleagues in a study that demonstrated a link between the clinical hemochromatosis phenotype and a human leukocyte antigen-A locus on chromosome 6p, with an inheritance pattern most consistent with an autosomal recessive disease.14 The specific gene causing most forms of hemochromatosis was discovered in 199615 and eventually named HFE, with the H shorthand for “high” and “FE” for iron. Subsequent studies identified cases of iron overload that were not caused by HFE mutations, leading to a gradual recognition of additional genes important in iron metabolism. The discovery of Hepcidin (coded by HAMP) by several groups in 200016 and 20013,17 eventually led to a unifying mechanism for iron genetic iron overload disease: impairment of hepcidin function.
Table 17.2 Summary of genetic iron diseases involving the liver | ||||||||||||||||||||||||||||||||||||||||||||||||||||||||||||
---|---|---|---|---|---|---|---|---|---|---|---|---|---|---|---|---|---|---|---|---|---|---|---|---|---|---|---|---|---|---|---|---|---|---|---|---|---|---|---|---|---|---|---|---|---|---|---|---|---|---|---|---|---|---|---|---|---|---|---|---|
|
hepatocytes, and Kupffer cells. Mutations in the HFE gene make the HFE protein unstable by disrupting disulfide bonds that are important for HFE binding to b2-microglobulin. The mutations also impair a key signaling pathway in hepcidin synthesis, known as the bone morphogenic protein signaling pathway.
Table 17.3 HFE mutations in northern European populations | ||||||||||||||||
---|---|---|---|---|---|---|---|---|---|---|---|---|---|---|---|---|
|
Table 17.4 HFE mutations in other populations | |||||||||||||||||||||||||
---|---|---|---|---|---|---|---|---|---|---|---|---|---|---|---|---|---|---|---|---|---|---|---|---|---|
|
including chronic viral hepatitis C and B, alcohol-related liver disease, nonalcoholic fatty liver disease (NAFLD), and α-1-antitrypsin deficiency. Although the data are incomplete and substantially mixed, the general trend suggests that HFE mutations predispose to iron accumulation in other chronic liver diseases and become increasingly penetrant as fibrosis progresses. As one example, cirrhotic livers with both α-1-antitrypsin deficiency and marked iron overload are enriched for HFE mutations.21 There are many negative studies that found no association between iron accumulation and fibrosis stage in other chronic liver diseases, most likely reflecting the difficulty of identifying a modest effect in the very complex setting of clinical cohort studies, where it is very difficult to adequately control for all of the factors that have been reported to influence both iron status as well as fibrosis risk.
descriptions are scant, but marked iron deposition is seen in both hepatocytes and Kupffer cells.46,47
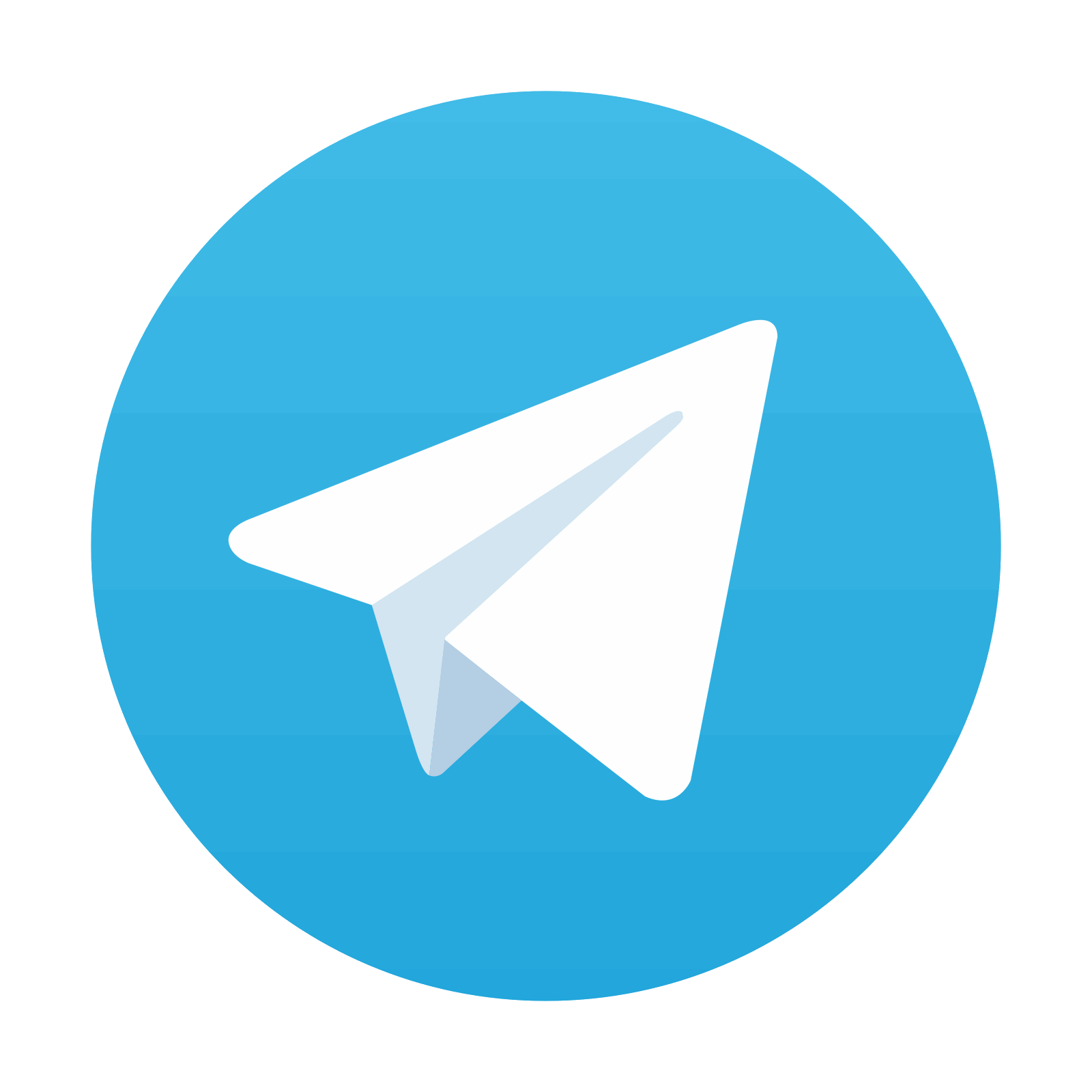
Stay updated, free articles. Join our Telegram channel

Full access? Get Clinical Tree
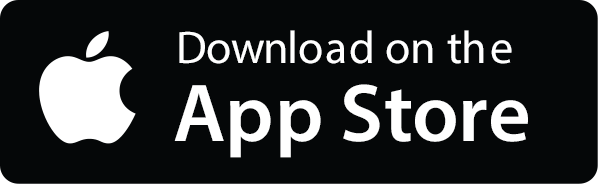
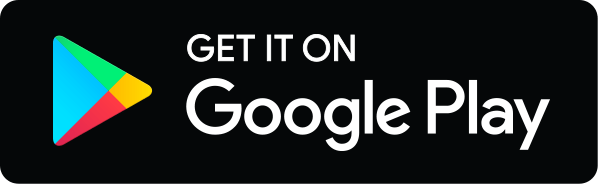