Introduction to the Immune System
Lori Broderick
Taylor A. Doherty
David Broide
I. INTRODUCTION
A. Role of the immune system. The primary role of the immune system is protection of self against continuously evolving pathogens. Normal immune function is characterized by recognition and elimination of microbial pathogens, tumors, and toxins without damage to the host. Imbalances in this complex system can result in either impaired host defense against infections or inappropriate host tissue damage (allergic disease, autoimmunity).
B. Innate and adaptive immune responses. The immune system can be divided into innate and adaptive components. The innate immune system is germline encoded and is evolutionarily more primitive. Activation of innate pattern-recognition receptors (PRRs) leads to rapid production of proinflammatory cytokines and activation of immune cells. Adaptive immunity is characterized by the development of antigen-specific primary and memory responses that occur through random somatic gene rearrangements. Dysfunction of either innate or adaptive responses may have catastrophic effects on the host, including susceptibility to infection (immune deficiency), autoimmunity (loss of self-tolerance), or hypersensitivity.
C. Physical barriers. The host-environment interface is made up of barriers, such as the skin and mucosal surfaces, which contain enzymes and mucus that inhibit the attachment of microbes or are directly antimicrobial. Ciliated surfaces, as are found in the respiratory tract, propel foreign substances out of the host. Additional physiologic barriers that allow the host to be inhospitable for some pathogens include body temperature elevation and low pH as is found in the stomach. Pathogens have evolved distinct mechanisms to overcome these barriers, and additional mechanisms of defense are required for the host to thrive.
II. DEVELOPMENT OF THE IMMUNE SYSTEM
Understanding the development of the immune system provides important insight into mechanisms contributing to B-cell and T-cell immunodeficiencies.
A. Immunity in the fetus and newborn. Human fetal development is a unique state in which a functional immune system develops under potential graft versus host conditions. At 4 to 5 weeks of gestation, the fetal liver acts as the primary site of lymphoid development, and subsequent lymphocyte production occurs in the bone marrow. The third and fourth pharyngeal pouches in the embryo give rise to the thymus, a lymphoid organ, which descends into the mediastinum by 7 weeks of gestation. The thymus is the site of T-cell
development and serves as a checkpoint for appropriate T-cell recognition of antigen and prevention of autoreactivity. From 6 to 8 weeks of gestation, large numbers of T-lymphocyte precursors migrate through the thymic layers, with the potential to become circulating immunocompetent T cells. Only about 5% of the developing T cells survive the stringent selection criteria, and those remaining undergo programmed cell death or apoptosis.
development and serves as a checkpoint for appropriate T-cell recognition of antigen and prevention of autoreactivity. From 6 to 8 weeks of gestation, large numbers of T-lymphocyte precursors migrate through the thymic layers, with the potential to become circulating immunocompetent T cells. Only about 5% of the developing T cells survive the stringent selection criteria, and those remaining undergo programmed cell death or apoptosis.
The human fetus is able to synthesize IgM antibody by 10.5 weeks of gestation, IgG by 12 weeks, and IgA antibody by 30 weeks. Due to the lack of antigen stimulation in utero, the immunocompetent newborn has little circulating IgA and IgM. IgG is nearly completely maternal in origin, delivered by active and selective transport across the placenta. The functional ability of fetal immunoglobulins is limited, with impaired switching to IgG and IgA and failure to respond to certain capsular polysaccharides. Serum levels of immunoglobulins, as well as their function, increase with aging (Table 1-1).
There is almost no placental transfer of complement components C1q, C2, C4, C3, and C5, and the total hemolytic complement in the newborn is low. These deficiencies may explain the relative impairment in opsonization
found in newborns, as the complement system does not functionally reach an adult level until 3 to 6 months of life. Phagocytic cells are seen in the human fetus at 8 weeks of gestation as myelocytes, and histiocytes are present in the early yolk sac stage of hematopoiesis. Monocytes first appear in the spleen and lymph nodes at 16 to 20 weeks of gestation, with gradual maturation of macrophage function with advanced fetal age.
found in newborns, as the complement system does not functionally reach an adult level until 3 to 6 months of life. Phagocytic cells are seen in the human fetus at 8 weeks of gestation as myelocytes, and histiocytes are present in the early yolk sac stage of hematopoiesis. Monocytes first appear in the spleen and lymph nodes at 16 to 20 weeks of gestation, with gradual maturation of macrophage function with advanced fetal age.
Table 1-1 Immunoglobulin (IgG, IgM, IgA, IgE) Serum Levels in the Fetus and Infant in the First Year of Life | ||||||||||||||||||||||||||||||||||||||||||||||||||||||||||||||||||
---|---|---|---|---|---|---|---|---|---|---|---|---|---|---|---|---|---|---|---|---|---|---|---|---|---|---|---|---|---|---|---|---|---|---|---|---|---|---|---|---|---|---|---|---|---|---|---|---|---|---|---|---|---|---|---|---|---|---|---|---|---|---|---|---|---|---|
|
B. Bone marrow development of B Cells. B cells are generated from multipotent hematopoietic stem cells (HSCs) in the bone marrow before migrating through the circulation to secondary lymphoid organs for further maturation (Fig. 1-1). The commitment to the B-cell lineage is first observed by expression of cell surface markers, such as CD19. The pro-B cells do not express immunoglobulin and undergo gene rearrangement prior to becoming mature B cells (Fig. 1-2). Antigen-inexperienced mature B cells express surface IgM or both IgM and IgD.
B cells are able to produce immunoglobulin with exquisite antigen specificity through a process of gene rearrangement. The immunoglobulin molecule has two identical heavy chains and two identical kappa or lambda light chains (Fig. 1-3). The chromosomal light and heavy chain loci are separated into multiple gene segments that code for the variable (V), diversity (D), joining (J), and constant (C) regions. The rearrangement of these genes is dependent on the lymphoid-specific recombinase activating gene proteins, RAG-1 and RAG-2, and terminal deoxynucleotidyl transferase (TdT). Rearrangement of first the heavy chain and then the light chain leads to assembly of a functional immunoglobulin with specificity for one antigen. The chains are formed by one of many V, D, and J genes recombining along with the addition of nucleotides at the joining ends of each gene cassette, a process known as junctional diversity. Further antibody diversity is created as one unique heavy chain unites with a similarly rearranged unique VJ light chain.
Surface immunoglobulin, referred to as the B-cell receptor, has a short cytoplasmic tail that associates with Iga and Igb and forms a complex that transduces intracellular signals upon antigen binding. The B-cell receptor
acts as a survival signal for the cell, and following initial recombination events, the naïve B cell expressing IgM and IgD enters the circulation and migrates to the secondary lymphoid organs. Hypermutation of the immunoglobulin genes takes place following encounter with antigen in the germinal centers of secondary lymphoid organs and leads to a process that increases antigen affinity, called affinity maturation. Switching the type of immunoglobulin from IgM to IgA, IgG, or IgE also occurs after antigen exposure and is termed isotype switching. B cells that develop into
immunoglobulin-secreting plasma cells are either short-lived in tissues or long-lived in bone marrow.
acts as a survival signal for the cell, and following initial recombination events, the naïve B cell expressing IgM and IgD enters the circulation and migrates to the secondary lymphoid organs. Hypermutation of the immunoglobulin genes takes place following encounter with antigen in the germinal centers of secondary lymphoid organs and leads to a process that increases antigen affinity, called affinity maturation. Switching the type of immunoglobulin from IgM to IgA, IgG, or IgE also occurs after antigen exposure and is termed isotype switching. B cells that develop into
immunoglobulin-secreting plasma cells are either short-lived in tissues or long-lived in bone marrow.
C. Thymic development of T cells. The thymus is the primary site of T-cell development and maturation and is divided into lobules, each with an outer cortex and an inner medulla. Thymocyte progenitors arrive from the bone marrow at the corticomedullary junction and migrate outward to the cortex. The stromal framework of the thymus contains epithelial cells, interdigitating with dendritic cells (DCs) and macrophages that assist in the development of T cells and removal of thymocytes that fail the selection process. The thymus atrophies with age, beginning at puberty, although T-cell development continues throughout life, albeit at a lower volume compared to that in childhood.
During T-cell development, mandatory recognition of foreign proteins in the context of self structures becomes an important checkpoint known as
selection (Fig. 1-4). Thymocytes begin as double-negative cells that do not express either CD4 or CD8 and begin the process of recombination to form a T-cell receptor (TCR). The TCR consists of αβ or γδ chains, which are formed by rearranging arrays of variable (V), diversity (D), and joining (J) regions similar to B cells. Rearrangement is dependent on RAG-1, RAG-2, and Artemis, which create the enormous combinatorial and junctional diversity of the T-cell repertoire. Loss of any of these enzymes is associated with a failure to generate T cells and a severe combined immunodeficiency (SCID) phenotype. Large gaps between gene cassettes result in the splicing out of double-stranded DNA that can circularize to form T-cell receptor excision circles, or TRECs, which have been introduced as a screening tool for SCID (see Chapter 20, Section VI. A: “Nucleotide-Based Testing”). Successful rearrangement of two TCR genes with surface expression of an αβ or γδ TCR marks the end of the pre-T-cell stage. More than 90% of T cells have rearranged the ab loci and will become double-positive T cells expressing both CD4 and CD8 and progress through further cell selection and differentiation. However, if the rearrangement of the γδ TCR is successful, the cells remain double negative and emigrate to lymphoid tissues and epithelial tissues.
selection (Fig. 1-4). Thymocytes begin as double-negative cells that do not express either CD4 or CD8 and begin the process of recombination to form a T-cell receptor (TCR). The TCR consists of αβ or γδ chains, which are formed by rearranging arrays of variable (V), diversity (D), and joining (J) regions similar to B cells. Rearrangement is dependent on RAG-1, RAG-2, and Artemis, which create the enormous combinatorial and junctional diversity of the T-cell repertoire. Loss of any of these enzymes is associated with a failure to generate T cells and a severe combined immunodeficiency (SCID) phenotype. Large gaps between gene cassettes result in the splicing out of double-stranded DNA that can circularize to form T-cell receptor excision circles, or TRECs, which have been introduced as a screening tool for SCID (see Chapter 20, Section VI. A: “Nucleotide-Based Testing”). Successful rearrangement of two TCR genes with surface expression of an αβ or γδ TCR marks the end of the pre-T-cell stage. More than 90% of T cells have rearranged the ab loci and will become double-positive T cells expressing both CD4 and CD8 and progress through further cell selection and differentiation. However, if the rearrangement of the γδ TCR is successful, the cells remain double negative and emigrate to lymphoid tissues and epithelial tissues.
Double-positive T cells in the thymic cortex undergo further selection events to ensure adequate recognition of antigen in the context of selfmajor histocompatibility complex (MHC) (Fig. 1-4). The T cells initially undergo positive selection in which double-positive T cells interact with self-MHC and self-peptide complexes expressed on cortical thymic epithelium. T cells that do not bind self-MHC undergo apoptosis and are cleared by macrophages. T cells that survive positive selection move to the medulla for negative selection. Negative selection ensures that T cells with high affinity for self-MHC and self-peptide are cleared generating central tolerance. The self-peptides expressed by thymic epithelial cells are governed by a gene known as the autoimmune regulator (AIRE). Mutations in AIRE are responsible for the disease autoimmune polyendocrinopathy-candidiasis-ectodermal dystrophy syndrome (APECED). During the selection process, double-positive T cells mature to either CD8+ T cells or CD4+ T cells on the basis of their interaction with either MHC class I (CD8+) or MHC class II (CD4+) molecules, respectively. Approximately 30% to 40% of circulating T cells are CD8+ and 60% to 70% are CD4+. Only about 5% of the developing T cells survive the selection events and enter the periphery.
Natural killer T cells (NKT) are another population of T cells that undergo a type of selection process in the thymus but recognize glycolipid presented on MHC-like molecules such as CD1d. NKT cells have an invariant TCR with limited antigen recognition and are largely considered innate cells with the capacity to rapidly produce large amounts of cytokines.
D. Secondary lymphoid tissues. The spleen, lymph nodes, and gut-associated lymphoid tissue (tonsils, adenoids, and Peyer’s patches) are secondary lymphoid organs and are organized to facilitate maximal interaction between B and T cells. Lymph nodes are dispersed throughout the body and function to localize the spread of infection. Lymph nodes are arranged in a reticular pattern with a cortex and medulla. B cells are primarily found in the cortex “B zone” (follicles and germinal centers), whereas T cells are primarily
found in the “T zone,” paracortical cords beneath the follicles. Circulating lymphocytes enter lymph nodes, tonsils, and Peyer’s patches from the blood by crossing high endothelial venules. These unique postcapillary venules display adhesion molecules, such as L-selectin, to facilitate the adhesion of lymphocytes and their migration into the lymphoid tissue. The spleen is also divided into T- and B-cell areas similar to that of the lymph node, but circulating lymphocytes enter from the blood through the marginal zone.
found in the “T zone,” paracortical cords beneath the follicles. Circulating lymphocytes enter lymph nodes, tonsils, and Peyer’s patches from the blood by crossing high endothelial venules. These unique postcapillary venules display adhesion molecules, such as L-selectin, to facilitate the adhesion of lymphocytes and their migration into the lymphoid tissue. The spleen is also divided into T- and B-cell areas similar to that of the lymph node, but circulating lymphocytes enter from the blood through the marginal zone.
III. INNATE IMMUNITY
A. Innate immune cells. The innate immune system is the first line of defense in protecting the host from invading pathogens and toxins. Deficiencies in innate immunity increase susceptibility to infection, while activation of innate inflammatory responses by allergens contribute to enhanced allergic symptoms. Innate immune cells including natural killer (NK) cells, NKT cells, macrophages, DCs, neutrophils, eosinophils, and mast cells contribute to initial inflammatory responses along with structural cells such as epithelial cells.
Natural killer (NK) cells, macrophages, and neutrophils play an important innate immune role in host defense against infections. NK cells are cytotoxic lymphocytes that act as a surveillance cell to detect viral-infected cells. Activated NK cells rapidly produce large amounts of interferon-gamma (IFN-g), which inhibits viral replication. NK cells are identified by their expression of CD56 and CD16 and are inhibited by encounter with self-MHC molecules.
Macrophages are phagocytic cells capable of producing a wide range of cytokines, significantly influencing the pro- or anti-inflammatory nature of the immune response. Macrophages are divided into subtypes and include M1 macrophages that promote Th1 responses through interleukin-12 (IL-12) and “alternatively activated” M2 macrophages induced by interleukin-4/ interleukin-13 (IL-4/IL-13) that promote Th2 responses and tissue remodeling. “M2-like” macrophages also exist and are activated by immune complexes.
Dendritic cells (DCs) are innate cells with a critical role in shaping adaptive responses to invading organisms or allergens. DCs play a key role in taking up antigen (or allergen) at mucosal or cutaneous surfaces and transporting the antigen to regional lymph nodes to encounter and expand populations of antigen-specific T cells. There are two general subtypes of DCs: the myeloid DCs and plasmacytoid DCs. Myeloid DCs are professional antigen-presenting cells with long-reaching dendritic extensions for sampling antigen and express high levels of CD11c and MHC class II. They are found in tissues and lymphoid structures. Plasmacytoid DCs are primarily found in tissues and are characterized by their plasmacytoid morphology. Plasmacytoid DCs express less MHC class II on their surface compared with myeloid DCs, thus limiting their ability to induce T-cell activation. Plasmacytoid DCs have an important role in innate immunity through production of type 1 interferons after toll-like receptor (TLR)-7 and TLR-9 stimulation.
Neutrophils are multilobed granulocytes that contain three classes of granule proteins: primary (azurophil) granules of myeloperoxidase, secondary (specific) granules of lactoferrin, and tertiary (gelatinase) granules of gelatinase. These are formed sequentially during granulocytic differentiation in the bone marrow. Neutrophils make up 50% to 70% of the circulating leukocytes.
Upon their emigration from the bone marrow, neutrophils respond to multiple chemokines including IL-8. They have important microbicidal activity, largely through their generation of oxygen radicals. Dysfunction of neutrophils is associated with severe susceptibility to infection, as seen in patients with neutropenia and chronic granulomatous disease (CGD).
Upon their emigration from the bone marrow, neutrophils respond to multiple chemokines including IL-8. They have important microbicidal activity, largely through their generation of oxygen radicals. Dysfunction of neutrophils is associated with severe susceptibility to infection, as seen in patients with neutropenia and chronic granulomatous disease (CGD).
Eosinophils are identified by their bilobed nucleus and specific granules. The Th2 cytokine IL-5 stimulates eosinophil development from CD34+ hematopoietic progenitor cells in the bone marrow. They represent 1% to 6% of leukocytes in circulation and migrate to sites of inflammation in response to eotaxin-1 and RANTES. Their survival depends on continued exposure to IL-5, as well as granulocyte macrophage colony-stimulating factor (GM-CSF), and IL-3. Eosinophils are effector cells in allergic responses and are important in antiparasitic inflammatory responses, in part through mediators such as major basic protein.
Mast cells are tissue resident cells derived from bone marrow progenitor cells. They play a key role in IgE-mediated immediate hypersensitivity reactions but can also be activated by non-IgE-mediated pathways. Two subsets of mast cells have been identified based on the contents of their secretory granules. Connective tissue-type mast cells (MCTC) are found in the skin, and their granules contain tryptase and chymase, whereas mucosaltype mast cells (MCT) contain tryptase but not chymase. Mast cells have surface IgE bound to the high-affinity IgE receptor (FcεR1). Antigen binding to surface IgE results in cross-linking and release of preformed mediators such as histamine, as well as synthesis of leukotrienes and a variety of cytokines including TNF-α, IL-3, IL-4, IL-5, IL-8, IL-10, IL-13, and MCP-1.
Basophils are granulocytes characterized by a lobulated nucleus and basophilic cytoplasmic granules containing histamine. Basophils represent <3% of leukocytes in the circulation and, like mast cells, have high-affinity receptors for IgE (FcεRI). They contribute to immediate and late-phase allergic reactions and are capable of generating cytokines including IL-4, IL-13, TSLP (see Section III D), and IL-25.
B. Phagocytosis. Phagocytosis is the process of engulfment of material by cells. Immune cells capable of phagocytosis include the circulating granulocytes (neutrophils and monocytes) and the cells of the reticuloendothelial system including liver Kupffer cells, splenic macrophages, pulmonary alveolar macrophages, and CNS microglial cells. Phagocytic cells are responsible for removal of microorganisms as well as apoptotic cells. Migration occurs through chemotaxis toward activated complement components (C3a, C4a, C5a), bacterial products, leukotrienes, and other mediators. Extracellular substances are recognized and internalized into a specific organelle known as a phagosome. The phagosome permits isolation and localization of the pathogenic material into an intracellular vacuole, separate from host cell functions, where metabolic activation can destroy the foreign material. NADPH oxidase generates superoxide anion radicals that are microbicidal and lead to an oxidative burst within the phagosome. Mutations in NADPH oxidase are responsible for chronic granulomatous disease (see Chapter 18, “Primary Immunodeficiency Diseases”), in which a defective oxidative burst leads to reduced pathogen killing by neutrophils and immunodeficiency.
C. Pattern-recognition receptors. PRRs are germline-encoded, innate danger signal sensors that detect invariant pathogen pattern motifs and initiate an
inflammatory response appropriate to the type of pathogen threat encountered. Diseases linked to PRRs include immunodeficiencies, inflammatory bowel disease, and hereditary autoinflammatory syndromes. There are two broad categories of recognized patterns, pathogen-associated molecular patterns (PAMPs) and damage-associated molecular patterns (DAMPs). PAMPs are microbial in origin, and DAMPs are nonmicrobial danger signals such as the presence of intracellular uric acid crystals in gouty arthritis. Recognition of both PAMPs and DAMPs by PRRs provide for the detection and response to a wide variety of “danger” signals. Four distinct subsets of PRRs have been identified, including TLRs, C-type lectin receptors (CLRs), nucleotide-binding oligomerization domain (NOD), leucinerich repeat containing receptors (NLRs), and retinoic acid-inducible gene I protein (RIG-I) helicase receptors.
inflammatory response appropriate to the type of pathogen threat encountered. Diseases linked to PRRs include immunodeficiencies, inflammatory bowel disease, and hereditary autoinflammatory syndromes. There are two broad categories of recognized patterns, pathogen-associated molecular patterns (PAMPs) and damage-associated molecular patterns (DAMPs). PAMPs are microbial in origin, and DAMPs are nonmicrobial danger signals such as the presence of intracellular uric acid crystals in gouty arthritis. Recognition of both PAMPs and DAMPs by PRRs provide for the detection and response to a wide variety of “danger” signals. Four distinct subsets of PRRs have been identified, including TLRs, C-type lectin receptors (CLRs), nucleotide-binding oligomerization domain (NOD), leucinerich repeat containing receptors (NLRs), and retinoic acid-inducible gene I protein (RIG-I) helicase receptors.
There are 13 mammalian TLRs, 10 of which have been identified in humans and are designated by extracellular domains containing leucine-rich repeats and cytoplasmic Toll/IL-1R homology (TIR) domains required to initiate signaling. TLRs act by forming homo- or heterodimers with other TLR family members, or heterodimers with other PRRs. TLRs are highly expressed on DCs, macrophages, and neutrophils and recognize bacteria, viruses, and fungi. For example, TLR4 recognizes LPS in the gram-negative bacterial cell wall, and TLR9 recognizes CpG motifs present in bacterial DNA. TLRs 1, 2, 4, 5, and 6 are located mainly on the cell surface, whereas TLRs 3, 7, 8, and 9 are found in endocytic compartments. TLR signaling molecules include interleukin-1 receptor-associated kinase 4 (IRAK4) deficiency and myeloid differentiation primary response gene 88 (MyD88). Deficiency of IRAK5 and MyD88 has recently been discovered as a cause of rare primary immunodeficiencies.
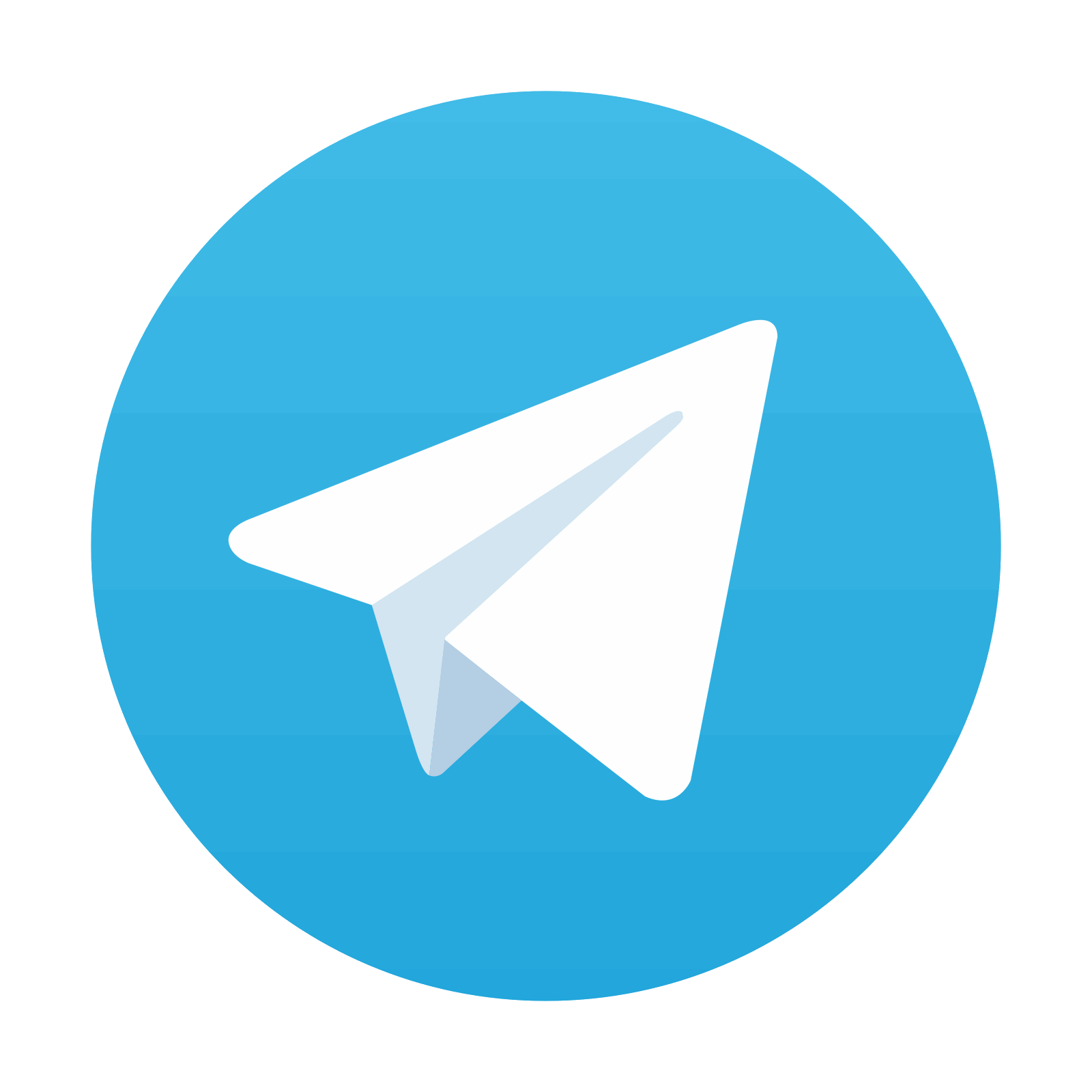
Stay updated, free articles. Join our Telegram channel

Full access? Get Clinical Tree
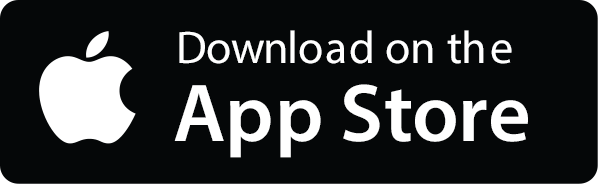
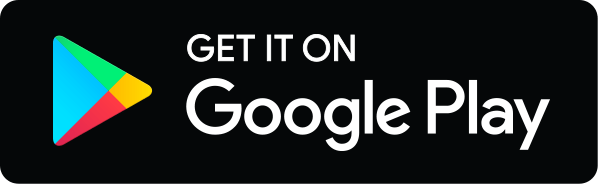