Objectives
- Contrast the terms endocrine, paracrine, and autocrine.
- Define the terms hormone, target cell, and receptor.
- Understand the major differences in mechanisms of action of peptides, steroids, and thyroid hormones.
- Compare and contrast hormone actions exerted via plasma membrane receptors with those mediated via intracellular receptors.
- Understand the role of hormone-binding proteins.
- Understand the feedback control mechanisms of hormone secretion.
- Explain the effects of secretion, degradation, and excretion on plasma hormone concentrations.
- Understand the basis of hormone measurements and their interpretation.
General Principles of Endocrine Physiology: Introduction
The function of the endocrine system is to coordinate and integrate cellular activity within the whole body by regulating cellular and organ function throughout life and maintaining homeostasis. Homeostasis, or the maintenance of a constant internal environment, is critical to ensuring appropriate cellular function.
The Endocrine System: Physiologic Functions and Components
- Regulation of sodium and water balance and control of blood volume and pressure
- Regulation of calcium and phosphate balance to preserve extracellular fluid concentrations required for cell membrane integrity and intracellular signaling
- Regulation of energy balance and control of fuel mobilization, utilization, and storage to ensure that cellular metabolic demands are met
- Coordination of the host hemodynamic and metabolic counterregulatory responses to stress
- Regulation of reproduction, development, growth, and senescence
In the classic description of the endocrine system, a chemical messenger or hormone produced by an organ is released into the circulation to produce an effect on a distant target organ. Currently, the definition of the endocrine system is that of an integrated network of multiple organs derived from different embryologic origins that release hormones ranging from small peptides to glycoproteins, which exert their effects either in neighboring or distant target cells. This endocrine network of organs and mediators does not work in isolation and is closely integrated with the central and peripheral nervous systems as well as with the immune systems, leading to currently used terminology such as “neuroendocrine” or “neuroendocrine-immune” systems for describing their interactions. Three basic components make up the core of the endocrine system.
The classic endocrine glands are ductless and secrete their chemical products (hormones) into the interstitial space from where they reach the circulation. Unlike the cardiovascular, renal, and digestive systems, the endocrine glands are not anatomically connected and are scattered throughout the body (Figure 1–1). Communication among the different organs is ensured through the release of hormones or neurotransmitters.
Figure 1–1.
The endocrine system. Endocrine organs are located throughout the body, and their function is controlled by hormones delivered through the circulation or produced locally or by direct neuroendocrine stimulation. Integration of hormone production from endocrine organs is regulated by the hypothalamus. ACTH, adrenocorticotropic hormone; CRH, corticotropin-releasing hormone; FSH, follicle-stimulating hormone; GHRH, growth hormone-releasing hormone; GnRH, gonadotropin-releasing hormone; LH, luteinizing hormone; MSH, melanocyte-stimulating hormone; TRH, thyrotropin-releasing hormone; TSH, thyroid-stimulating hormone; T3, triiodothyronine; T4, thyroxine.
Hormones are chemical products, released in very small amounts from the cell, that exert a biologic action on a target cell. Hormones can be released from the endocrine glands (ie, insulin, cortisol); the brain (ie, corticotropin-releasing hormone, oxytocin, and antidiuretic hormone); and other organs such as the heart (atrial natriuretic peptide), liver (insulin-like growth factor 1), and adipose tissue (leptin).
Hormone Chemistry and Mechanisms of Action
Based on their chemical structure, hormones can be classified into proteins (or peptides), steroids, and amino acid derivatives (amines). Hormone structure, to a great extent, dictates the location of the hormone receptor, with amines and peptide hormones binding to receptors in the cell surface and steroid hormones being able to cross plasma membranes and bind to intracellular receptors. An exception to this generalization is thyroid hormone, an amino acid–derived hormone that is transported into the cell in order to bind to its nuclear receptor. Hormone structure influences the half-life of the hormone as well. Amines have the shortest half-life (2–3 minutes), followed by polypeptides (4–40 minutes), steroids and proteins (4–170 minutes), and thyroid hormones (0.75–6.7 days).
Protein or peptide hormones constitute the majority of hormones. These are molecules ranging from 3 to 200 amino acid residues. They are synthesized as preprohormones and undergo post-translational processing. They are stored in secretory granules before being released by exocytosis (Figure 1–2), in a manner reminiscent of how neurotransmitters are released from nerve terminals. Examples of peptide hormones include insulin, glucagon, and adrenocorticotropic hormone (ACTH). Some hormones in this category, such as the gonadotropic hormone, luteinizing hormone, and follicle-stimulating hormone, together with thyroid-stimulating hormone (TSH) and human chorionic gonadotropin, contain carbohydrate moieties, leading to their designation as glycoproteins. The carbohydrate moieties play important roles in determining the biologic activities and circulating clearance rates of glycoprotein hormones.
Figure 1–2.
Peptide hormone synthesis. Peptide hormones are synthesized as preprohormones in the ribosomes and processed to prohormones in the endoplasmic reticulum (ER). In the Golgi apparatus, the hormone or prohormone is packaged in secretory vesicles, which are released from the cell in response to an influx of Ca2+. The increase in cytoplasmic Ca2+ is required for docking of the secretory vesicles in the plasma membrane and for exocytosis of the vesicular contents. The hormone and the products of the post-translational processing that occurs inside the secretory vesicles are released into the extracellular space. Examples of peptide hormones are adrenocorticotropic hormone (ACTH), insulin, growth hormone, and glucagon.
Steroid hormones are derived from cholesterol and are synthesized in the adrenal cortex, gonads, and placenta. They are lipid soluble, circulate bound to binding proteins in plasma, and cross the plasma membrane to bind to intracellular cytosolic or nuclear receptors. Vitamin D and its metabolites are also considered steroid hormones. Steroid hormone synthesis is described in Chapters 5 and 6.
Amino acid–derived hormones are those hormones that are synthesized from the amino acid tyrosine and include the catecholamines norepinephrine, epinephrine, and dopamine; as well as the thyroid hormones, derived from the combination of 2 iodinated tyrosine amino acid residues. The synthesis of thyroid hormone and catecholamines is described in Chapters 4 and 6, respectively.
Depending on where the biologic effect of a hormone is elicited in relation to where the hormone was released, its effects can be classified in 1 of 3 ways (Figure 1–3). The effect is endocrine when a hormone is released into the circulation and then travels in the blood to produce a biologic effect on distant target cells. The effect is paracrine when a hormone released from 1 cell produces a biologic effect on a neighboring cell, which is frequently a cell in the same organ or tissue. The effect is autocrine when a hormone produces a biologic effect on the same cell that released it.
Figure 1–3.
Mechanisms of hormone action. Depending on where hormones exert their effects, they can be classified into endocrine, paracrine, and autocrine mediators. Hormones that enter the bloodstream and bind to hormone receptors in target cells in distant organs mediate endocrine effects. Hormones that bind to cells near the cell that released them mediate paracrine effects. Hormones that produce their physiologic effects by binding to receptors on the same cell that produced them mediate autocrine effects.
Recently, an additional mechanism of hormone action has been proposed in which a hormone is synthesized and acts intracellularly in the same cell. This mechanism has been termed intracrine and has been identified to be involved in the effects of parathyroid hormone–related peptide in malignant cells and in some of the effects of androgen-derived estrogen (see Chapter 9).
Hormones released into the circulation can circulate either freely or bound to carrier proteins, also known as binding proteins. The binding proteins serve as a reservoir for the hormone and prolong the hormone’s half-life, the time during which the concentration of a hormone decreases to 50% of its initial concentration. The free or unbound hormone is the active form of the hormone, which binds to the specific hormone receptor. Thus, hormone binding to its carrier protein serves to regulate the activity of the hormone by determining how much hormone is free to exert a biologic action. Most carrier proteins are globulins and are synthesized in the liver. Some of the binding proteins are specific for a given protein, such as cortisol-binding proteins. However, proteins such as globulins and albumin are known to bind hormones as well. Because for the most part these proteins are synthesized in the liver, alterations in hepatic function may result in abnormalities in binding-protein levels and may indirectly affect total hormone levels. In general, the majority of amines, peptides, and protein (hydrophilic) hormones circulate in their free form. However, a notable exception to this rule is the binding of the insulin-like growth factors to 1 of 6 different high-affinity binding proteins. Steroid and thyroid (lipophilic) hormones circulate bound to specific transport proteins.
The interaction between a given hormone and its carrier protein is in a dynamic equilibrium and allows adjustments that prevent clinical manifestations of hormone deficiency or excess. Secretion of the hormone is adjusted rapidly following changes in the levels of carrier proteins. For example, plasma levels of cortisol-binding protein increase during pregnancy. Cortisol is a steroid hormone produced by the adrenal cortex (see Chapter 6). The increase in circulating levels of cortisol-binding protein leads to an increased binding capacity for cortisol and a resulting decrease in free cortisol levels. This decrease in free cortisol stimulates the hypothalamic release of corticotropin-releasing hormone, which stimulates ACTH release from the anterior pituitary and consequently cortisol synthesis and release from the adrenal glands. The cortisol, released in greater amounts, restores free cortisol levels and prevents manifestation of cortisol deficiency.
As already mentioned, the binding of a hormone to a binding protein prolongs its half-life. The half-life of a hormone is inversely related to its removal from the circulation. Removal of hormones from the circulation is also known as the metabolic clearance rate: the volume of plasma cleared of the hormone per unit of time. Once hormones are released into the circulation, they can bind to their specific receptor in a target organ, they can undergo metabolic transformation by the liver, or they can undergo urinary excretion (Figure 1–4). In the liver, hormones can be inactivated through phase I (hydroxylation or oxidation) and/or phase II (glucuronidation, sulfation, or reduction with glutathione) reactions, and then excreted by the liver through the bile or by the kidney. In some instances, the liver can actually activate a hormone precursor, as is the case for vitamin D synthesis, discussed in Chapter 5. Hormones can be degraded at their target cell through internalization of the hormone-receptor complex followed by lysosomal degradation of the hormone. Only a very small fraction of total hormone production is excreted intact in the urine and feces.
Figure 1–4.
Hormone metabolic fate. The removal of hormones from the organism is the result of metabolic degradation, which occurs mainly in the liver through enzymatic processes that include proteolysis, oxidation, reduction, hydroxylation, decarboxylation (phase I), and methylation or glucuronidation (phase II) among others. Excretion can be achieved by bile or urinary excretion following glucuronidation and sulfation (phase II). In addition, the target cell may internalize the hormone and degrade it. The role of the kidney in eliminating hormone and its degradation products from the body is important. In some cases urinary determinations of a hormone or its metabolite are used to assess function of a particular endocrine organ based on the assumption that renal function and handling of the hormone are normal.
Hormone Cellular Effects
The biologic response to hormones is elicited through binding to hormone-specific receptors at the target organ. Hormones circulate in very low concentrations (10–7 – 10–12 M), so the receptor must have high affinity and specificity for the hormone to produce a biologic response.
Affinity is determined by the rates of dissociation and association for the hormone-receptor complex under equilibrium conditions. The equilibrium dissociation constant (Kd) is defined as the hormone concentration required for binding 50% of the receptor sites. The lower the Kd, the higher the affinity of binding. Basically, affinity is a reflection of how tight the hormone-receptor interaction is. Specificity is the ability of a hormone receptor to discriminate among hormones with related structures. This is a key concept that has clinical relevance as will be discussed in Chapter 6 as it pertains to cortisol and aldosterone receptors.
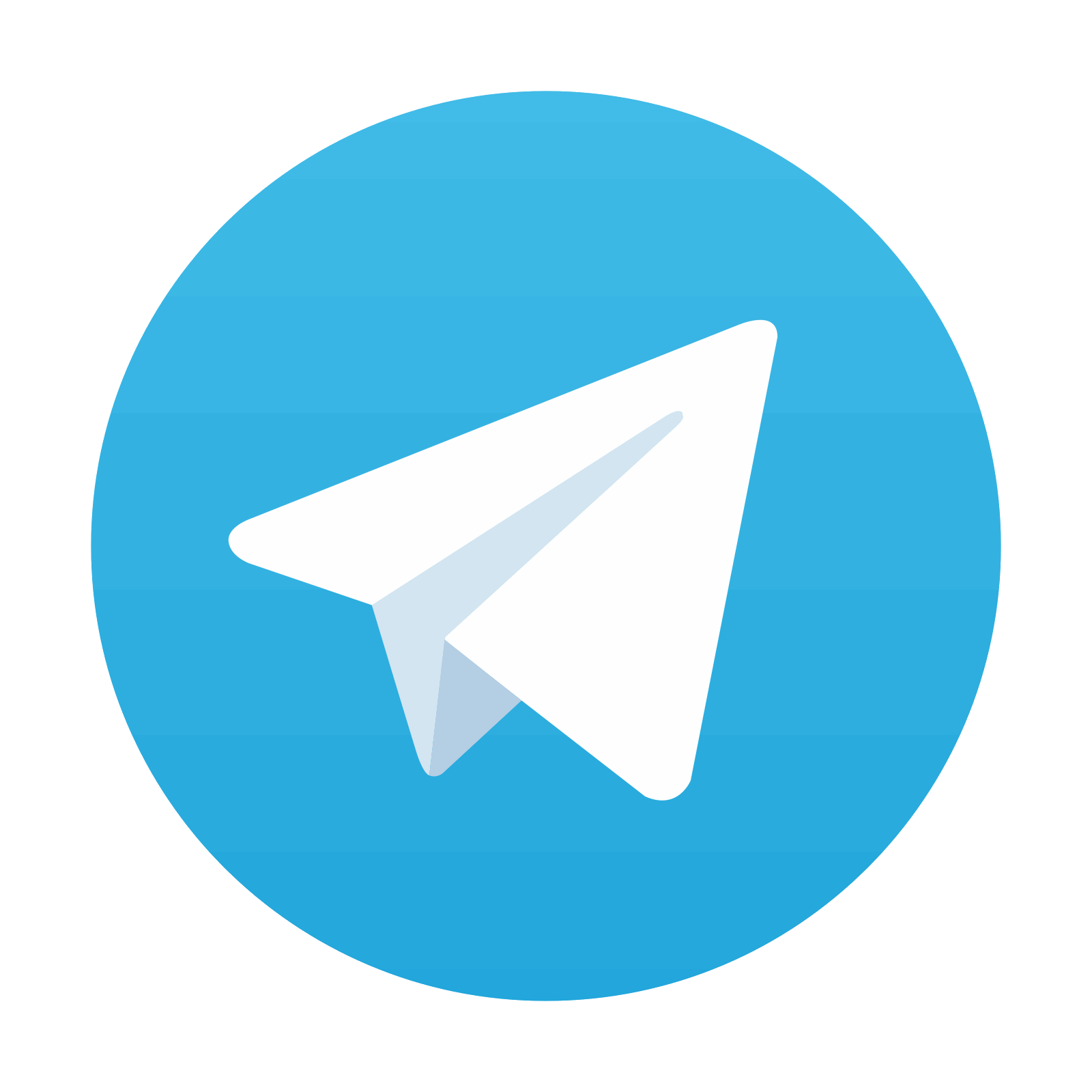
Stay updated, free articles. Join our Telegram channel

Full access? Get Clinical Tree
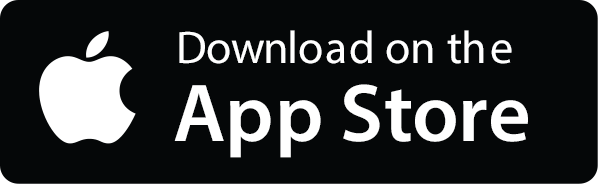
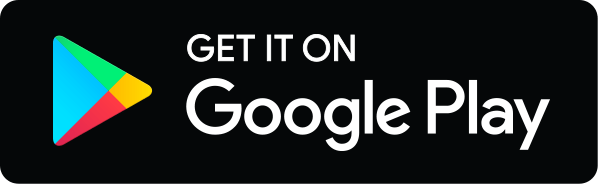