Gastric ACID Secretion
One of the unique properties of the mammalian stomach is its ability to secrete large quantities of 0.16 N hydrochloric acid. This secretory process represents one element in an elaborate system that allows higher organisms to regulate their food intake. Gastric acid secretion represents the outcome of several regulatory signals. Secretion can be initiated by a wide variety of factors related to the ingestion of food and the caloric status of the individual. In most current texts of physiology, the regulation of acid secretion is considered to occur in “phases,” which were previously termed the cephalic, gastric, and intestinal phases and believed to function in an overlapping manner in the regulation of acid secretion.
The terms implied that individual regulatory mechanisms originated in the central nervous system (CNS) (cephalic phase) or in the periphery (gastric and intestinal phases). Because our current understanding of such mechanisms recognizes that both the central and peripheral components function as parts of an overlapping and integrated process, the use of the term phase has become inaccurate. The central regulation of acid secretion involves the cortical and spinal cord structures; peripheral regulation is defined as neural, endocrine, and paracrine pathways. In this section, we initially discuss the general principles of regulation of gastric acid secretion by the brain, nerves, and endocrine or paracrine cells. Thereafter, we detail the transmitters and their effects on individual cells. Finally, we outline regulation at the level of the parietal cell.
The complexity of neural, endocrine, and paracrine regulation is illustrated in the following table, which displays ligands that have been shown to affect gastric acid secretion by direct action on gastric mucosal cells as well as their probable cellular site of action.
There is, therefore, a remarkable number of ligands used to control acid secretion in the gastric mucosa, showing that the process of digestion of food has been refined over the course of evolution to a remarkably exact system.
Receptors on Gastric Cells | |||||
---|---|---|---|---|---|
Cell Type | |||||
Receptor | ECL | G | D | Parietal | Chief |
CCK1 | No | No | Yes | No | Yes |
CCK2 | Yes | Yes | Yes | Yes | No |
PACAP | Yes | ? | VIP | ? | Yes |
M1, M3, M5 | No | Yes | No | Yes | Yes |
M2, 4 | No | No | Yes | No | No |
GRP | No | Yes | Some | ? | ? |
SST | Yes | Yes | Yes | Yes | Yes |
Ca | ? | Yes | ? | ? | ? |
Y1 | Yes | ? | ? | ? | ? |
Gal | Yes | ? | ? | ? | ? |
Histamine | H3 | No | H3 | H2 | Species |
CGRP | No | No | Perhaps | No | No |
Amino acids | No | Yes | No | No | No |
pH | No | Perhaps | Perhaps | No | No |
The various ligands that have been shown to affect functions of isolated gastric cells. All of these also affect acid secretion in vivo, but understanding of their pathways has required studies on isolated cells, glands, and organs. |
Central regulation
The CNS, particularly via the vagus, is responsible for the initiation of acid secretion. Although it is well recognized that the sight, smell, taste, or thought of food can stimulate acid secretion, it is less appreciated that hypoglycemia itself comprises virtually the strongest central stimulus for acid secretion. Thus, insulin is a powerful stimulant, and its effect is mimicked by 2-deoxyglucose, which, by forming 2-deoxyglucose-6-phosphate, effectively acts to inhibit glucose metabolism in the regulatory centers of the CNS.
There are two crucial events necessary for stimulation of acid secretion: activation of the parietal cell and increased blood flow. The stimulation of blood flow depends on the release of nitric oxide (NO) from the endothelial cells in the gastric vasculature, and this may be due to stimulation by calcitonin gene-related peptide (CGRP) or by histamine acting at an H2 receptor. Activation of the parietal cell depends on vagal stimulation of the enteric nervous system (ENS). All the interneuronal connections in the ENS are muscarinic, because adequate doses of atropine ablate acid secretion induced by sham feeding or even thyroid-releasing hormone (TRH) central injection. H2 antagonists in the rat can block only 60% of acid secretion due to sham feeding; therefore, pathways
other than those impinging on the ECL cell account for 40% of central stimulation of acid secretion.
other than those impinging on the ECL cell account for 40% of central stimulation of acid secretion.
There are several regions within the CNS responsible for detecting and transmitting central stimuli. The dorsomotor nucleus of the vagus (DMNV), the hypothalamus, and the nucleus tractus solitarius (NTS) have been identified as central structures that are key participants in the regulatory process. The final integration of central stimuli appears to occur in the DMNV, which supplies stimulatory efferent fibers to the stomach via the vagus nerve. Its destruction eliminates central stimulation of acid secretion, whereas electrical stimulation results in a strong secretory response. The DMNV appears to function as a central integrator of function and does not appear to initiate stimulation itself but rather integrates central sensory input that arises primarily from the hypothalamus or as visceral sensory input from the NTS. Vagal efferents to the stomach arise also from the nucleus ambiguus (NA), but these appear to be primarily involved in regulating motility rather than secretion.
In the hypothalamus, several sites have been identified as exerting stimulatory and inhibitory influences on acid secretion. The ventromedial hypothalamus (VMH) appears to exert a tonic inhibitory influence, because its ablation enhances secretion, whereas electrical stimulation suppresses secretion. The VMH appears to function by inhibiting the stimulatory signals arising from the lateral hypothalamus (LH) and adjacent medial forebrain bundle (MFB). The latter two structures mediate the response to the hypoglycemic stimulation of acid secretion. Both direct and indirect connections from the LH and MFB to the DMNV have been identified.
The NTS also responds directly to glucose deprivation and initiates stimulation of acid secretion mediated via the DMNV. In addition to the glucose deprivation response, the NTS also receives other major neural inputs that include taste fibers and visceral afferents, which presumably participate in the modulation of the secretory process. The former probably initiate acid secretion related to taste. Visceral afferent input to the NTS arises primarily from synapses in the inferior ganglion of the vagus. Greater than 95% of vagal neural fibers are afferent rather than efferent. This means that visceral sensory input to the CNS plays an important part in the continuous central modulation of gastric function and is necessary to assure integration of CNS and peripheral mechanisms.
Sensory information from the stomach is relayed to the CNS by both vagal afferent fibers and sympathetic afferent fibers. The sensory receptors of the stomach consist primarily of unmyelinated nerve endings that detect mechanical (distention and touch), chemical, and thermal stimuli. Receptive fields for the
sympathetic afferents appear to lie mainly within the smooth muscle layers and surrounding blood vessels. Despite this location, the sensory fibers are more sensitive to chemical (e.g., bradykinin) than to mechanical stimulation. The recognition that bradykinin is a mediator of inflammatory responses suggests that these sympathetic afferents encode painful sensations associated with gastritis and ulcers. This concept was initially recognized by Wolff and Wolff in their observations of Tom, a patient with a permanent gastric fistula. Except for major distention, the normal stomach is relatively insensitive to mechanical and chemical stimuli, at least in terms of pain. On the other hand, inflamed regions of the mucosa are quite sensitive to the application of chemicals and even light touch. It is thus likely that the pain associated with gastritis may arise from the release of inflammatory mediators or the sensitization of sympathetic sensory fibers. In this respect, the observation that the sympathetic receptors that respond to bradykinin also respond to capsaicin, the irritant component of cayenne pepper, may be of relevance. Capsaicin is a sensory neurotoxin that exerts its effects by opening calcium channels in the plasma membrane of sensory nerve endings. This first results in stimulation of the nerve, but prolonged application leads to degeneration of the nerve fiber and loss of sensation.
sympathetic afferents appear to lie mainly within the smooth muscle layers and surrounding blood vessels. Despite this location, the sensory fibers are more sensitive to chemical (e.g., bradykinin) than to mechanical stimulation. The recognition that bradykinin is a mediator of inflammatory responses suggests that these sympathetic afferents encode painful sensations associated with gastritis and ulcers. This concept was initially recognized by Wolff and Wolff in their observations of Tom, a patient with a permanent gastric fistula. Except for major distention, the normal stomach is relatively insensitive to mechanical and chemical stimuli, at least in terms of pain. On the other hand, inflamed regions of the mucosa are quite sensitive to the application of chemicals and even light touch. It is thus likely that the pain associated with gastritis may arise from the release of inflammatory mediators or the sensitization of sympathetic sensory fibers. In this respect, the observation that the sympathetic receptors that respond to bradykinin also respond to capsaicin, the irritant component of cayenne pepper, may be of relevance. Capsaicin is a sensory neurotoxin that exerts its effects by opening calcium channels in the plasma membrane of sensory nerve endings. This first results in stimulation of the nerve, but prolonged application leads to degeneration of the nerve fiber and loss of sensation.
Vagal afferent fibers are found in the smooth muscle layers and the mucosa of the stomach. The receptors in the muscle layers are primarily tension or stretch receptors capable of detecting motility changes. Although these receptors detect and regulate motility of the muscle layers, they also are involved in the vagovagal reflexes (the so-called long reflexes) associated with distention-dependent secretory activity, an important element in the peripheral regulation of acid secretion.
Neural regulation of acid secretion
The primary function of the peripheral neural regulatory mechanisms is to modulate and integrate the stimuli (histamine and gastrin) that act directly on the parietal cell itself. This section, therefore, addresses the known neuronal factors involved in regulation of the parietal cell, directly or indirectly.
Cholinergic
The efferent fibers of the vagus nerve do not innervate the parietal cells directly but synapse with ganglion cells of the ENS. It is thus likely that the CNS serves to modulate the ENS regulatory mechanisms, because there exists a great numeric disparity between efferent vagal fibers and ganglia of the ENS. Approximately 2,000 vagal fibers synapse with an estimated 10 million ganglia, suggesting that, rather than exerting any direct control of parietal cell function, an intermediate class of modulators exists.
The majority of the cell bodies of enteric neurons are found in the two plexuses, the myenteric (Auerbach’s plexus) and the submucosal (Meissner’s plexus). Because the enteric plexuses of the stomach have not been studied in as much detail as those of the small and large intestines, a description of the organization and function of the gastric enteric neurons relies often on information gleaned from other segments of the gastrointestinal tract.
The myenteric plexus lies between the circular and longitudinal layers of smooth muscle and is primarily associated with coordination of motility. The
submucosal neurons supply nerve fibers directly to the mucosal cells, as well as to the loosely arranged smooth muscle cells contained within the submucosal layer. The submucosal ganglia receive a variety of synaptic inputs, which include projections from the myenteric plexus as well as extrinsic nerve fibers that consist primarily of postganglionic sympathetic fibers.
submucosal neurons supply nerve fibers directly to the mucosal cells, as well as to the loosely arranged smooth muscle cells contained within the submucosal layer. The submucosal ganglia receive a variety of synaptic inputs, which include projections from the myenteric plexus as well as extrinsic nerve fibers that consist primarily of postganglionic sympathetic fibers.
An important feature of the ENS, as with other components of the autonomic nervous system, is that the postganglionic nerve fibers are polymodal, releasing two or more neurotransmitters. Individual nerve fibers may thus contain both a conventional neurotransmitter [e.g., acetylcholine (ACh) or norepinephrine] and one or more neuropeptides, such as pituitary adenylate cyclase-activating peptide (PACAP), galanin, or calcitonin gene-releasing peptide (CGRP). Whether both types of transmitter are released simultaneously or differentially is unclear, but this configuration provides opportunity for a single nerve fiber to engender a number of diverse responses. Another feature of many postganglionic nerve fibers of the ENS is that transmitter release can occur along an extended length of the nerve axon. This reflects the existence of periodic swellings or varicosities along the axon that are assumed to be the sites for transmitter release. Postganglionic nerve fibers and epithelial cells of the stomach do not exhibit conventional synapses, usually evident at the neuromuscular junction, although some nerve fibers terminate near the mucosal cells. This spatial organization suggests that transmitters are released into the extracellular space and diffuse to nearby cells, where they may act if appropriate cellular receptors are present. The advantage of regional rather than conventional synapses is activation of several cells but possibly a number of divergent cell types, as well as obviation of the formation of new synapses as the epithelial or endocrine cells are replaced.
ACh exhibits a number of different actions when it is released from postganglionic nerve fibers in the fundic mucosa. Regional release of ACh activates parietal cells directly by binding to an M3-subtype muscarinic receptor. In the gastric antrum, ACh is able to stimulate all the G cells to secrete gastrin. This hormone is conveyed via the systemic circulation to the fundus. At this site, gastrin activates the ECL cell via a gastrin/cholecystokinin (CCK2) receptor to release histamine. In the antrum, ACh also inhibits the release of somatostatin from D cells, which removes a tonic inhibition of gastrin release and thus indirectly augments acid secretion by elevation of gastrin.
Adrenergic
There is some evidence that the sympathetic fibers in the gastric mucosa are involved in regulation of blood flow and perhaps also are able to stimulate ECL cell release of histamine. In general, sympathetic innervation appears to play a minor role in regulation of gastric function.
Peptidergic pathways
A variety of neuropeptides have been shown to affect acid secretion, such as gastrin-releasing peptide (GRP), CGRP, galanin, and PACAP. Some of these are targeted to one or another of the gastric endocrine cells. A problem that has arisen in interpretation of in vivo data is that both activating and inhibitory peptides can be released at the same time, thus confounding analysis of possible physiologic consequences of their release.
Gastrin-releasing peptide
GRP is a member of the subfamily of peptides that terminate with Leu-Met-NH2 and is 27 amino acids in length. Immunoreactivity is found in nerves but not in endocrine cells in the intestine, but it is also found in endocrine cells in the stomach. Vagal stimulation increases GRP in the venous outflow of the stomach, and GRP antibodies or antagonists reduce gastrin release, whether caused by vagal stimulation or peptone meals. There is, therefore, good evidence that GRP is an important noncholinergic neural mediator of gastrin secretion. The release of somatostatin that is observed after GRP administration may be indirect, perhaps due to the effect of the elevated gastrin on the fundic D cell. Hence, it is not surprising that the maximal effect of GRP on acid secretion is found in the lower regions of the dose-response curve, because at higher levels, somatostatin release probably predominates. GRP stimulates Ca2+ signaling in an isolated G cell preparation. It might be expected that GRP would stimulate fundic but not antral D cells.
Calcitonin gene-related peptide
CGRP is a 37-residue peptide with a disulfide bridge and a C-terminal amide. CGRP immunoreactive afferent nerve fibers are found in the gastric myenteric plexus, muscle, submucosal blood vessels, and mucosa. The peptide inhibits gastric acid secretion, whether given centrally or peripherally. The latter is probably due to stimulation of somatostatin release; no effect has been found in isolated rat ECL cells. Inhibition of acid secretion by injected CGRP must be due to effects on either antral or fundic D cells, or both.
Galanin
Galanin is a 29-amino acid peptide found in nerve endings in the gastric mucosa that inhibits basal- and pentagastrin-stimulated acid secretion. There are three isoforms of the galanin receptor, one of which apparently stimulates and two of which inhibit calcium signaling. Galanin inhibits gastrin release but also inhibits gastrin-stimulated acid secretion. It does not inhibit bethanechol- or histamine-stimulated acid secretion. It therefore has an additional site of action distal to the G cell. Galanin interferes with stimulation of the ECL cell by a pertussis toxin (PTX)sensitive pathway. This peptide has dual sites of inhibitory effect—both the G and ECL cells.
PACAP
PACAP is the newest member of the vasoactive intestinal peptide (VIP)/glucagon/secretin family. It is released from nerves, although their location in the gastrointestinal tract is not known. The PACAP receptor exists as several splice variants, and PACAP stimulates Ca2+ signaling in pancreatic acinar cells, showing that this receptor is coupled to both adenylate cyclase and phospholipase C. PACAP stimulates calcium signals and histamine release from the ECL cell. Several variants of this receptor have been shown to be present by reverse transcriptase-polymerase chain reaction (RT-PCR) of an ECL cell cDNA library.
Whereas injection of PACAP inhibits acid secretion, when this is done in the presence of antisomatostatin antibody, stimulation of acid secretion results. D cells have VIP receptors; hence, injection of PACAP will nonselectively release somatostatin. Local neural release will result in ECL cell stimulation and acid secretion. VIP nerves are presumably those that stimulate the D cell. PACAP
also stimulates ECL cell growth as effectively as gastrin. Presumably, its release is acute, that of gastrin chronic.
also stimulates ECL cell growth as effectively as gastrin. Presumably, its release is acute, that of gastrin chronic.
The CCK2 and the PACAP receptors appear to be functionally the most important in terms of stimulation of histamine release from the ECL cell—the former endocrine, the latter neural.
Vasopressin
This peptide also inhibits gastric acid secretion, but only in the intact animal. Its action is exerted on gastric mucosal blood flow. On stimulation, there is a large increase in mucosal perfusion, and this is essential for normal acid secretion. If blood flow is impeded, with consequent relative hypoxia of the parietal cell, acid secretion is also inhibited.
Paracrine regulation
Histamine
Initially, it was proposed that histamine is released from mucosal mast cells, but more recent studies indicate that gastric histamine is released from a specialized endocrine cell of the stomach, the ECL cell. The release of histamine from the ECL cell is regulated by various factors involving neural, endocrine, paracrine, and autocrine pathways, such as PACAP and galanin.
Somatostatin
The peptide is released from both fundic and antral D cells. The latter are more numerous. Somatostatin release is used almost universally as a downregulator of cellular function and, therefore, although a peptide, must have a short half-life to ensure organ specificity. This allows classification of this inhibitor as a paracrine mediator of acid secretion.
Endocrine regulation
Gastrin
Gastrin is the major stimulatory peptide for histamine release from the ECL cell. It is present in the G cells of the antral gland, in approximately the midregion in the human stomach. It is released from the G cell in response to aromatic amino acids or amines in the gastric lumen and in response to cholinergic stimulation or GRP released from postganglionic fibers of the vagus nerve. Acidification of the gastric lumen inhibits gastrin release. Although its effect on gastric acid secretion has been relegated to a secondary effect by stimulation of histamine release from the ECL cell, gastrin remains the major endocrine regulator of gastric acid secretion. Changes in circulating gastrin account for much of the gastric response to feeding.
Cholecystokinin
The peptide is present in both the pancreas and duodenum and has a broad spectrum of activities. Of particular interest in terms of gastric physiology is its ability to stimulate the D cell and the chief cell of the gastric mucosa at high
affinity on a CCK1 receptor. It is approximately equally as effective as gastrin in stimulation of ECL cell Ca2+ signaling and histamine release, but the CCK1 octapeptide (nonsulfated) does not result in growth stimulation of the ECL cell in vitro.
affinity on a CCK1 receptor. It is approximately equally as effective as gastrin in stimulation of ECL cell Ca2+ signaling and histamine release, but the CCK1 octapeptide (nonsulfated) does not result in growth stimulation of the ECL cell in vitro.
Peptide YY
Peptide YY (PYY) was first isolated from the intestine. PYY and neural peptide (NPY) interact with three receptor subtypes: Y1, Y2, and Y3. PYY is released by meals, and relatively large doses have been shown to inhibit gastrin-stimulated acid secretion. This peptide may be a component of enterogastrone, a complex group of factors released during the intestinal phase of digestion, resulting in inhibition of gastric acid secretion. The ECL contains the Y1-receptor subtype, and histamine release and calcium signaling are inhibited by PYY.
Enterogastrone
Gastric inhibitory peptide (GIP) and glucagon-like peptide (GLP) are thought to be components of the factors released during the intestinal phase of digestion that inhibit acid secretion. Relatively high doses of GIP and GLP inhibited gastrin-induced signaling and histamine release from ECL cells.
Secretin
A complex feedback mechanism exists communicating to the stomach from the intestine. Secretin inhibits gastric acid secretion, presumably in part by stimulation of the D cell. However, it also inhibits, at least in some species, histamine-stimulated acid secretion, indicating a direct action on the parietal cell.
Control of gastric acidity
The interaction of the triumvirate of the ECL cell, G cell, and D cell serves to regulate the release of histamine, as illustrated in the simplified model of the figure.
Because histamine, together with ACh and possibly gastrin, is responsible for the activation of the parietal cell, and somatostatin, epidermal growth factor (EGF), galanin, and secretin inhibit the parietal cell, the secretion of acid represents an example of neural, endocrine, and paracrine positive and negative interactions. The overall goal of these processes is to regulate the acidity of the gastric contents. The regulatory mechanisms must be able to detect the intragastric pH and respond with celerity and accuracy.
The only known mechanism that at this time corresponds to such a requirement is the suppression of gastrin release by pH less than 3.0. The low pH may, in fact, be detected by the antral D cell and then release of somatostatin that results in the suppression of gastrin secretion. This relatively simple feedback loop between acid secretion and gastrin release appears to function in vivo, as indicated by the observation that disruption of the loop by antisecretory agents, such as H2 receptor antagonists or pump inhibitors, culminates in hypergastrinemia. Although the pH dependence of gastrin secretion represents the major known mechanism for control of gastric acidity, it is unlikely that so simple a feedback loop can account fully for the maintenance of gastric pH. Other mechanisms must be present, if only to regulate those types of acid secretion that are independent of gastrin (e.g., due to direct ACh stimulation of the parietal cell).
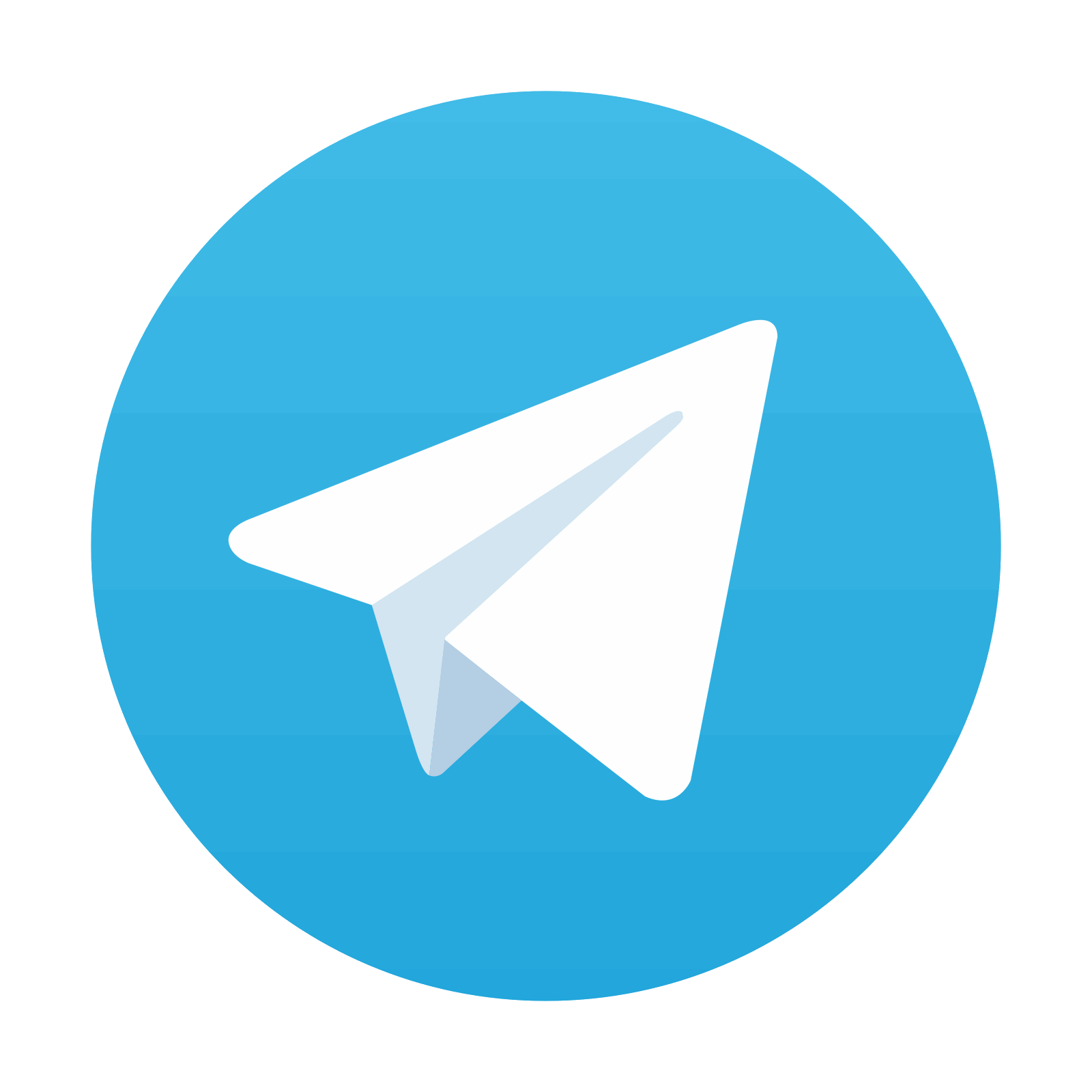
Stay updated, free articles. Join our Telegram channel

Full access? Get Clinical Tree
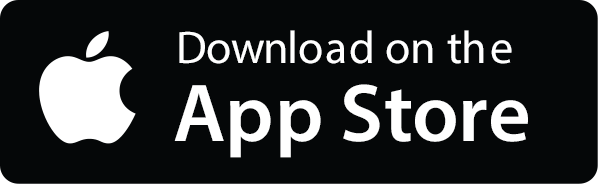
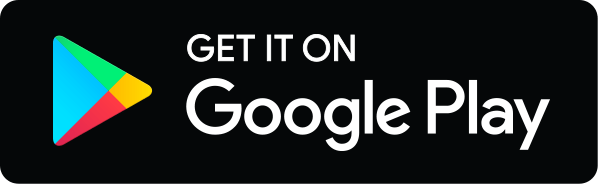