catecholamines are found with variation in the relative proportions in the peripheral nerves and adrenal medulla. For example, in amphibians, E is the predominant adrenergic neurotransmitter in distinction to mammals that utilize NE at sympathetic neuroeffector junctions. In mammals, for practical purposes, E is limited to the central nervous system (CNS) and to chromaffin cells of the adrenal medulla. (The term “chromaffin” is a portmanteau word derived from chromium and affinity; chromaffin cells darken markedly on exposure to dilute solutions of chromium salts.) Catecholamines, therefore, are widely distributed in nature, phylogenetically ancient, and it may thus be inferred that they act as signaling molecules throughout the animal kingdom.
destined to become chromaffin cells invade the anlagen of the developing adrenal cortex. Bone morphogenetic proteins derived from the dorsal aorta play a role in the differentiation of the neural crest cells into SA precursors. Nerve growth factor(s) derived from innervated tissues are involved in the development and maintenance of the sympathetic nervous system (SNS). Although the adrenal cortex is not required for the differentiation of adrenal medullary chromaffin cells, it is necessary for the synthesis of E, accomplished by the induction of the E-forming enzyme in the cytoplasm of chromaffin cells. In the sympathetic nerves innervating sweat glands, a phenotypic change in neurotransmitter from NE to acetylcholine (Ach) occurs during development, an example of the plasticity that exists in the developing nervous system.
TABLE 1.1 Landmark Discoveries in Catecholamine Research: Structure of First Circulating Hormone (Epinephrine); First Evidence for Both Neurochemical Transmission and for Specific Cell Surface Receptors; and Termination of Action by Uptake into the Sympathetic Nerve Endings | ||
---|---|---|
|
TABLE 1.2 Landmark Discoveries in Catecholamine Research: Signal Transduction; Subcellular Storage and Release by Exocytosis; Second Messengers; G-proteins; Reversible Protein Phosphorylation in Receptor Desensitization and Enzyme Activation | |||
---|---|---|---|
|
TABLE 1.3 Biologically Important Catecholamines in Humans: Epinephrine, Norepinephrine, Dopamine | ||
---|---|---|
|
Preganglionic neurons in the intermediolateral cell column of the spinal cord synapse with postganglionic sympathetic neurons in the sympathetic ganglia. Note that the adrenal medulla is analogous to the postganglionic sympathetic nerves; it receives a preganglionic cholinergic innervation from the splanchnic nerves and releases E into the circulation. Nerves that utilize Ach as their neurotransmitter are referred to as cholinergic; those utilizing NE are called adrenergic, per the suggestion of Sir Henry Dale in the 1930s (Table 1.6).
TABLE 1.4 Somatic and Autonomic Nervous Systems | ||||||||||||||||||
---|---|---|---|---|---|---|---|---|---|---|---|---|---|---|---|---|---|---|
|
example, are all controlled from the CNS by the ANS. The sympathetic branch of the ANS is also structured to promptly address external threats to the integrity of the organism, by supporting the organism for “fight or flight,” as described by Cannon early in the 20th century. Each preganglionic neuron of the SNS synapses with many postganglionic neurons in the sympathetic ganglia (average 1:20 pre to postganglionic cells), including neurons in ganglia above or below the level at which the preganglionic neuron exits the neuraxis. The adrenal medulla, furthermore, secretes E into the circulation thereby supporting the function of the SNS. That said, it is important to recognize that the SNS functions continuously
in the regulation of normal physiology and that this regulation is characterized by discriminating rather than generalized responses.
activity is associated with a decrease in PSNS outflow and vice versa. The actions stimulated by SNS and the PSNS are also antagonistic. Pulse rate, for example, is increased by the SNS and decreased by the PSNS. Gut motility and secretion, similarly, are suppressed by the SNS and stimulated by the PSNS. This dual control of autonomic functions permits more precise regulation in the maintenance of homeostasis than would be possible with a unidirectional system.
TABLE 1.5 Anatomic Organization of the Sympathetic and the Parasympathetic Nervous Systems | ||||||||||
---|---|---|---|---|---|---|---|---|---|---|
|
TABLE 1.6 Chemical Neurotransmission in the Autonomic Nervous System: Adrenergic and Cholinergic Nerves | ||||||||||||
---|---|---|---|---|---|---|---|---|---|---|---|---|
|
of NE is carried out in peripheral adrenergic nerves and in central neurons that utilize NE as a neurotransmitter. In the chromaffin cells of the adrenal medulla, and certain neurons of the CNS, NE is N-methylated to E.
ascorbate as a hydrogen donor; it is not substrate specific for DA, so it may hydroxylate a variety of phenylethylamines. The subcellular localization of DBH to the storage granules means that the final step in NE synthesis occurs within the storage site. DBH is both a structural component of the granule wall as well as a soluble component of the granule contents. The latter is released along with NE or E during SA activation, a fact accounting for the short-lived, and long gone, interest in plasma DBH as a marker of SA activity. Granular localization of DBH protects newly formed DA from degradation by cytoplasmic MAO.
face of increased SNS activity is demonstrated by the depletion in tissue NE when TH is inhibited.
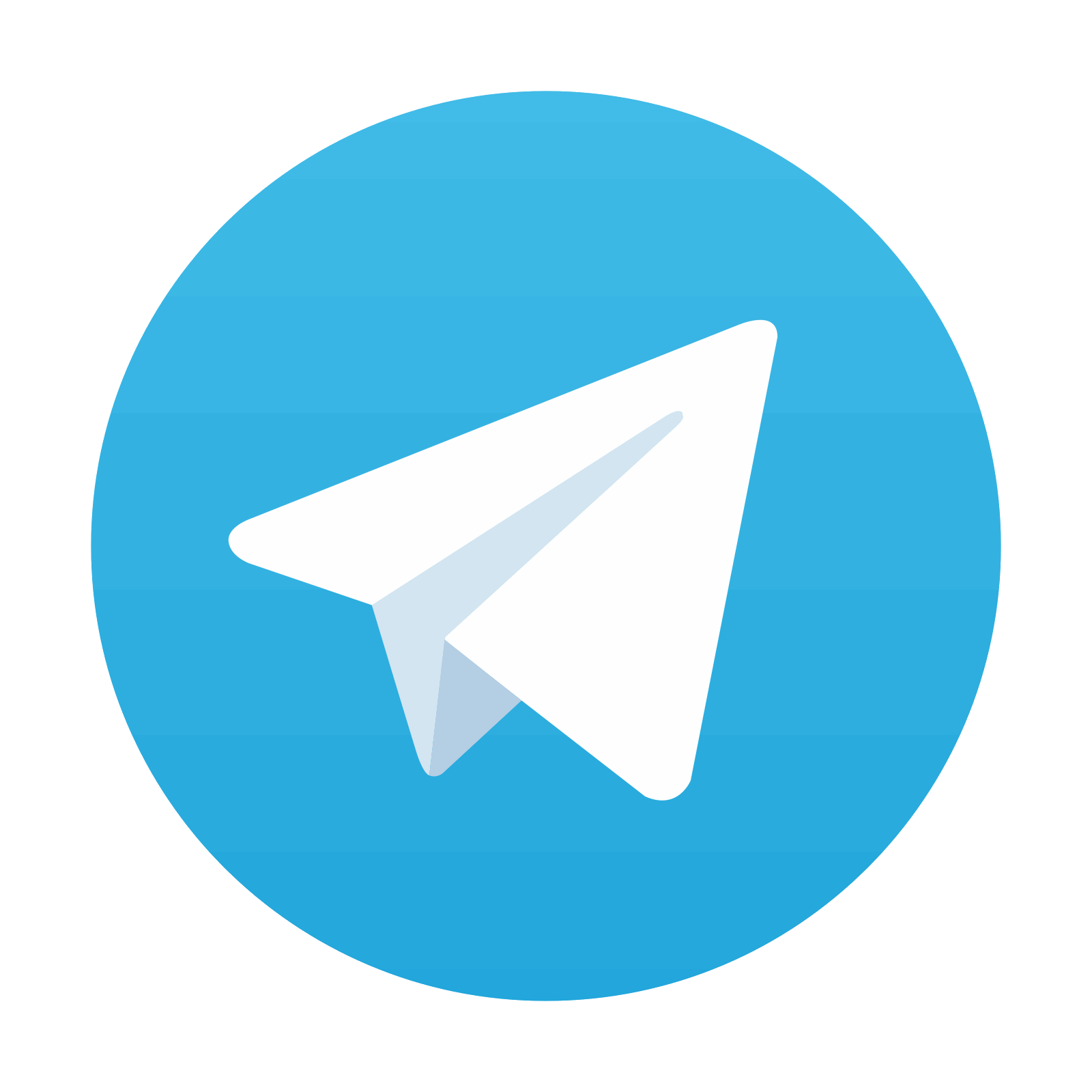
Stay updated, free articles. Join our Telegram channel

Full access? Get Clinical Tree
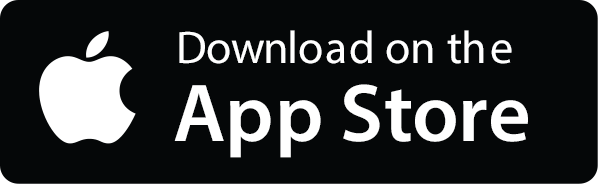
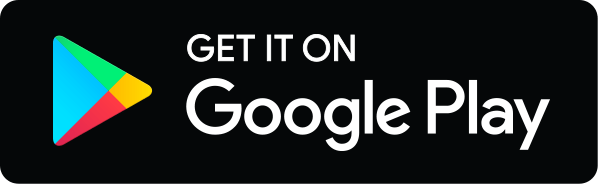