Flow Cytometric Analysis in Hematopathology Diagnosis
Gerard Lozanski
Alejandro A. Gru
INTRODUCTION
Multicolor flow cytometric analysis (FCA) is routinely used in diagnostic hematopathology. FCA allows for rapid and accurate characterization of the immunophenotype of a large number of cells in a variety of samples, thus providing essential data for accurate classification of hematopoietic malignancies. This chapter will briefly describe the basic technical concept of flow cytometric analysis, explain the limitations and potential pitfalls of this method, and provide a general outline of flow cytometric markers that are currently used in the diagnosis of hematopoietic malignancies, with particular focus on cutaneous lymphoproliferative disorders.
FLOW CYTOMETRIC ANALYSIS INSTRUMENTATION
Flow cytometric analyzers are instruments that are designed to measure (metry) properties of cells (cyto) that flow suspended in liquid media (flow). Flow cytometric instruments comprise a fluidics system, optic system, signal detection system, and a computer equipped with software that controls instrument functions, data acquisition, and data analysis.
Fluidics
The fluidics system is a network of pumps, valves, tubes, and flow cell, which are all designed to deliver a single file of cells to the interrogation point in the flow chamber. The single filing of the cells is achieved by hydrodynamic focusing where a suspension of cells (sample stream) is injected into the center of a stream of sheath fluid flowing through a specially designed, funnel-like, flow chamber that produces laminar flow. The shape of flow cell and differences in pressures between sheet fluid (outer layer) and sample stream (centrally located) are optimized to assure that the diameter of the sample stream is no wider than the single cell it contains, forcing cells to flow in a single file through the center of the interrogation point (Fig. 7-1).
Optics
The optic system comprises a light source, light “handling system,” and a detection system.
Light Sources
Current clinical flow cytometry (FC) instruments use low-power lasers and/or laser diodes as light sources. The light generated by these sources is monochromatic (of exact single wavelength/color) and coherent (all waves of light are parallel), allowing for the generation of a very fine light beam of desired intensity. Modern FC analyzers are often equipped with three or more lasers, allowing for the generation of several different wavelength beams that can simultaneously excite a large number of different fluorochromes. The point at which the light beam(s) intersects with the stream of cells within the flow chamber is called the interrogation point (Fig. 7-1). As expected, the precise alignment of the laser beam with the sample stream is critical for optimal performance of a flow cytometer.
Light Handling System
The interaction of the light beam with each cell in the sample stream, at the interrogation point, results in light scatter. In addition, if a cell was stained with a fluorochrome(s), the laser beam will induce fluorescence. Both scattered light (at the wavelength of the laser) and fluorescent light (wavelength specific for fluorochrome) are emitted at 360 degrees in a spherical fashion. To increase sensitivity and light collection efficiency, all modern flow cytometers’ light handling system comprising lenses, dichroic mirrors, and band-pass filters is linked to the flow chamber at the interrogation point via a network of optic fibers. Through this system, light signals from each cell are selectively distributed to an array of photo detectors (Fig. 7-2). The photo detectors convert light into electrical current that is proportional to the intensity of light signal for each cell. By adjusting the voltage of each photo-element, their light measuring sensitivity can be tuned up or down to assure optimal signal capture for each cell. The color of light that is delivered to a given photo detector is dictated by band-pass filters placed in the light path leading to a given detector. As a result, each detector can measure only a single color of light and is blinded to the rest of light signal generated by the analyzed cell. This combination of optic fiber, band-pass filters, and an array of detectors allows measurement of simultaneously emitted multicolored signals from each analyzed cell. Measured light is transformed into quantifiable separate color information. As a result, depending on the FC type, up to 12 different antigens (colors) can be measured on each cell at once in a highly precise and quantitative fashion.
COMPUTER SYSTEM
Current FC instruments are operated via complex software packages that include instrument operation software that controls fluidics, light source, and detection systems’ voltages and signal compensation. All raw data from each sample are saved in a highly standardized universal format known as list mode data. Stored list mode data allow for future reanalysis with adjustments to gating strategy and certain parameters such as compensation. List mode data are also very useful for comparison between specimens from different samples for the same patient, for purposes such as detection of minimal residual disease. A separate part of the software is designed for daily instrument quality assurance (QA) monitoring that assures optimal performance of fluidics, light, and detection systems. Part of this program keeps track of daily QA activities and performance. Each instrument is also equipped with software that keeps track of sample demographics, sample acquisition, data analysis, and data reporting. In general, each major FC manufacturer provides software packages optimized for their instrument. Such packages are often Food and Drug Administration (FDA)-approved and depending on specific application may require the use of kits of reagents and flow protocol. The best examples of such kit software instrument FDA-approved combinations are T-cell subsets and stem cell quantification packages. For interpretative FCA in hematopathology, the rules are less strict, and as long as proper test validation is performed, laboratories may use a combination of methods, reagents, and software of their choice.
CELL CHARACTERISTICS MEASURED BY FLOW CYTOMETRIC ANALYSIS
Intrinsic Cell Properties
Several cell characteristics can be measured without the need for additional reagents. Forward scatter (FS) and side scatter (SS) characteristics that measure cell size and cell complexity, respectively, are two intrinsic properties of cells routinely measured during FC analysis.
Forward Scatter
When a cell flows through the interrogation point and is hit by the light beam, it produces light beam absorption, reflection, diffraction, and scatter at a 360-degree angle. A photo-element that is placed in the direct path of the laser beam is charged with a collection of light scattered along the axis of the laser beam, so-called forward scatter light. FS light intensity is proportional to the cell size; a small cell produces a low FS signal and a large cell produces a strong FS signal. For example, lymphocytes that are small will produce a low FS signal, and monocytes/macrophages that are large in size will produce a strong FS signal (Fig. 7-3).
Side Scatter
Different types of cells show differences in complexity of cell surface and internal structures. These differences are reflected by the amount of scattered light that can be measured by a photo-element that is placed at a 90-degree angle in relation to the laser beam. Light beam interaction with complex cells such as hairy cells (extensive ruffling of cell surface) or granulocytes (complex internal structures) will scatter intensely and produce a strong SS signal. In contrast, a light beam interacting with low-complexity cells such as lymphocytes scatters less intensely and produces a low SS signal (Fig. 7-3).
The combination of FS and SS parameters helps to stratify a mixed population of cells into subsets representing red blood cells, lymphocytes, monocytes, granulocytes, and platelets with good accuracy (Fig. 7-3).
Extrinsic Cell Properties
Cell parameters that can be measured by FCA with use of additional reagents such as monoclonal antibodies, DNA-binding dyes, cytoplasmic dyes, and other more specialized dyes are termed extrinsic cell properties.
Immunophenotype Characterization
The addition of antigen-specific monoclonal antibodies (and in some cases, polyclonal antibodies) is the extrinsic property most commonly measured in clinical FCA.
The development of monoclonal antibodies that specifically recognize unique epitopes on antigens expressed on cell surface, antigens in the cell cytoplasm, and antigens in the nuclei allows for the detailed characterization of the antigenic makeup of each cell. Monoclonal antibodies are conjugated to different fluorochromes. The fluorochromes are dyes, which when excited with laser light rapidly absorb laser light energy and emit light with lower energy that is specific for a given fluorochrome. Thus, a single laser can simultaneously induce fluorescence from a different combination of dyes that will produce several distinct fluorescence signals. These signals are then captured by designated photo-elements that recognize only a single color of fluorescent light and are “blinded” for all other colors. Photo-elements transform the intensity of captured light into a digital signal that is proportional to the intensity of fluorescence emitted by each individual cell passing through the interrogation point. For example, helper T cells express CD3 and CD4 antigens and are negative for the expression of CD19 and CD13 antigens. When these cells are stained with a combination of antibodies for CD3 FITC (green dye), CD4 PE (yellow dye), CD19 CY5 (red dye), and CD13 CY7 (magenta dye), they will produce a positive signal for CD3 (green) and CD4 (yellow) and will have a negative signal for CD19 CY5 (red) and CD13 CY7 (magenta). As expected, B cells will be positive for CD19 CY5 (red signal) and will be negative for the remaining antigens and myeloid cells will be positive for CD13 CY7 (magenta signal) and will be negative for the rest of antigens noted above. Based on this principle and using different laser/dye/antibody combinations, a current clinical FC instrument can simultaneously characterize up to 12 fluorescence signals for each cell passing through the interrogation point, allowing for high-density characterization of a cell’s immunophenotype. With the use of special methods to permeabilize the cell membrane and the nuclear membrane, this technique is also used to evaluate cytoplasmic (MPO) and nuclear antigens (TDT, KI67). A modern flow cytometric instrument can analyze more than 10, 000 cells per second providing a very sensitive and robust method for determining the antigenic expression of a specific cell population.
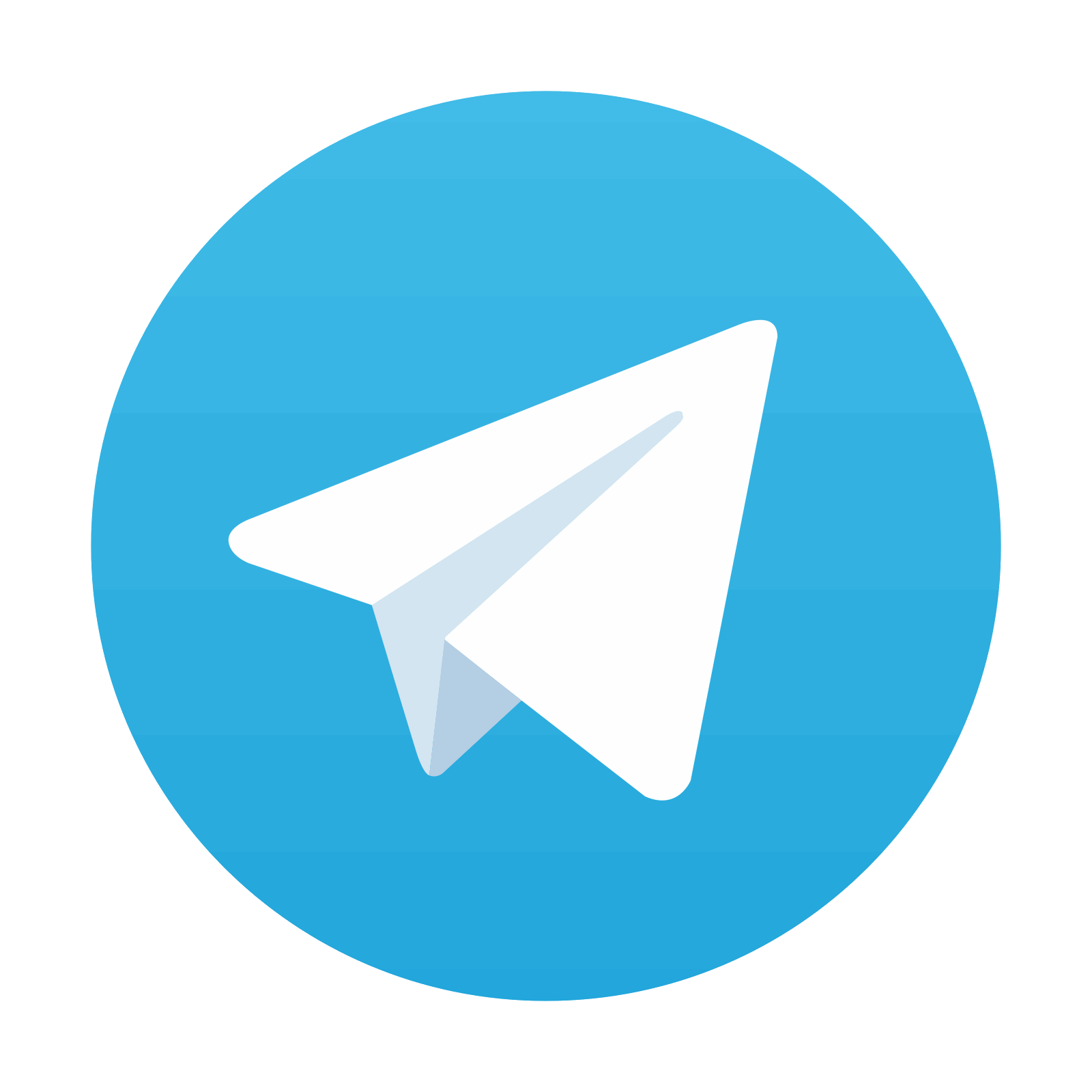
Stay updated, free articles. Join our Telegram channel

Full access? Get Clinical Tree
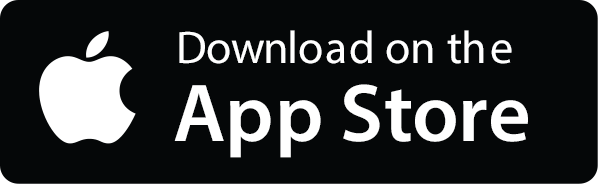
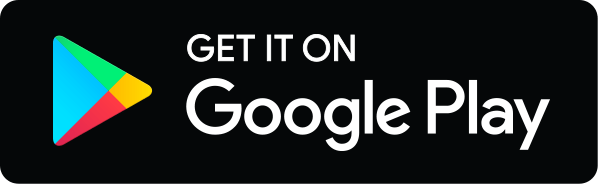