Evasion of Innate Host Defenses by SARS-CoV-2 and Its Pathogenetic Correlates
Roberto Patarca
William A. Haseltine
INTRODUCTION
Severe acute respiratory syndrome coronavirus 2 (SARS-CoV-2) has evolved multiple and redundant ways in which to evade and suppress antiviral innate immunity, thereby often leading the race between microbe and host by increasing human-to-human spread and readily infecting multiple animals, which can reinfect humans. The fact that not uncommonly more than one SARS-CoV-2 protein simultaneously antagonize distinct components of innate immune pathways underlies the evolution of CoVs into pathogens of medical and veterinary importance and underscores the need to further characterize host defenses against SARS-CoV-2 and other viruses. This knowledge informs development of preventive and therapeutic interventions and diagnostic approaches.
Twenty-six of the 29 polyfunctional accessory, nonstructural, and structural proteins synthesized by SARS-CoV-2 in infected cells are involved passively or actively, and locally to systemically, in blocking antiviral innate and adaptive immunity. Only the nonstructural protein (NSP7 and NSP8) members of the replication complex and the 13-amino-acid-long intrinsically disordered NSP11 have thus far not been linked to immune evasion. This large antihost defense arsenal shields the virus from host immunity and allows it to replicate and spread within the host and to other hosts. Viral countermeasures to the host cell’s fortification of outer defenses, boosting of internal defenses, self-destruction via autophagy, signaling other cells about the danger, and preventing newly synthesized viral particles from leaving the infected cell are seen at every stage of the virus life cycle from viral entry to exit from the host cell and its spread to other cells and hosts.
The seven accessory proteins (open reading frames [ORFs] 3a, 3b, 6, 7a, 7b, 8, and 9b) encoded in the distal third of the SARS-CoV-2 genome are involved in adaptation to specific hosts and in antagonizing interferon (IFN) production, inhibiting IFN signaling, and enhancing IFN resistance, among other mechanisms such as epigenetic regulation to evade host immune responses, including the production of proinflammatory cytokines, induction of autophagy, and major histocompatibility complex (MHC)-driven presentation of antigens. Although expression of ORFs 9c and 10 remains a matter of debate, there is evidence on immune-evasive activities in cell culture experiments and studies in an in vivo infection setting are warranted.
All structural proteins (nucleocapsid, envelope, membrane, and spike) also encoded in the distal third of the genome control its dissemination while regulating the immune response and contributing to the formation of double membrane vesicles and related structural compartments to conceal the replication machinery to avoid triggering cellular alarm systems.
Most of the NSPs (NSP1-NSP16), including the RNA-directed RNA polymerase, helicase, proteases, and others required for genome replication and encoded in the proximal two-thirds of the genome, have immune-evasive activities by interfering with host protein synthesis and cleavage, including those needed for autophagy, messenger RNA (mRNA) stability, bidirectional mRNA nucleocytoplasmic transport, and protein trafficking including nuclear or cell membrane localization, among other functions. Some viral NSPs cap the 5´ ends of genomic and subgenomic viral RNAs with a structure similar to that used by eukaryotic cells, which allows them to be perceived as endogenous rather than invader molecules, thereby evading degradation and triggering of host immune defenses. Others like NSP1 allow the virus to hijack the host cell translation machinery by inhibiting ribosome interactions with host mRNA but not viral RNAs, which are protected by NSP1 binding to stem-loop 1 (SL1) in their 5´-untranslated region (UTR). This affects translation of antiviral proteins while maintaining enough host cell protein translation for cell fitness.
This chapter reviews the IFN response, which is among the first lines of innate host defense against microbial infections, and how each of the SARS-CoV-2 accessory, nonstructural, and structural proteins and SARS-CoV-2 microRNAs contribute to evasion and suppression of the IFN response and other host defenses, such as natural killer (NK) cells. This immune evasion may also lead to the exaggerated inflammatory response underlying pathogenesis of severe coronavirus disease 2019 (COVID-19) and inform its prevention and treatment.
OVERVIEW OF THE INTERFERON RESPONSE IN INNATE IMMUNITY
The IFN response is among the first lines of innate host defense against microbial infections.1 IFN cytokines are grouped into IFN-I (IFN-α, -β, -ε, -ω, and -κ), IFN-II (IFN-γ), and IFN-III (IFN-λ) families. IFN-I-mediated immune response represents the first line of host defense against viral infection.2 IFN-I induces antiviral activity in cells to eliminate viral replication by inducing double-stranded RNA-activated kinase (Protein kinase R, PKR), 2´,5´-oligoadenylate synthetase (OAS), and ribonuclease (RNase) L.3, 4 and 5 The type I IFN family has complex functions throughout a microbial infection, acting initially mostly in the recruitment of immune cells to promote activation of the adaptive host immune response, inhibit proliferation of infected cells, and limit viral replication.6 The type II IFN family participates in macrophage and neutrophil activation, and its absence results in, for instance, increased virus replication and decreased survival of mice infected with herpes simplex virus type 2.7 IFN-III and IFN-I share many activities; however, IFN-III is more proapoptotic than IFN-I or -II.8
Host pattern recognition receptors (PRRs), including Toll-like (TLRs), retinoic acid–inducible gene I (RIG-I)-like (RLRs), cytoplasmic DNA, and nucleotide-binding and oligomerization domain (NOD)-like (NLRs) receptors, recognize viral pathogen-associated molecular patterns (PAMPs), such as single- and double-stranded RNAs,9, 10, 11, 12, 13 and 14 and activate IFN regulatory factors (IRF) 3 and 7 and their translocation to the cellular nucleus, where they bind IFN promoters triggering IFN production to extents that differ by cell type, with plasmacytoid dendritic cells likely secreting most of the IFN-I during SARS-CoV-1 infection.15,16
For RLRs, recognition of cognate ligands, such as viral RNA, triggers exposure of the RIG-I caspase activation and recruitment domain (CARD), which interacts with that of the mitochondrial antiviral adaptor protein (mitochondrial antiviral signaling protein [MAVS]) or myeloid differentiation primary response 88 (MyD88).6,17 MAVS/MyD88 activation recruits downstream signaling components to the mitochondria, thereby activating the inhibitor of κ-B kinase ε (IKKε) and TANK-binding kinase 1 (TBK1), which in turn phosphorylate IRF3 and IRF7. Phosphorylated IRF3 forms a dimer that translocates to the nucleus where it activates IFN-I gene transcription.18,19
In autocrine and paracrine manner, binding of secreted IFN-I (ie, IFN-α and IFN-β) and -III to their respective receptors on various cell types activates the Janus kinase 1 (JAK1) and Tyrosine kinase 2 (TYK2), which phosphorylate signal transducer and activator of transcription (STAT1 and STAT2) proteins20 that associate with IRF9 to form the IFN-stimulated gene (ISG) factor 3 (ISGF3) complex, which ultimately binds to IFN-stimulated response element (ISRE) promoters, resulting in the expression of hundreds of ISGs with antiviral functions.21,22 Likewise, IFN-II signaling results in STAT1 homodimer formation and production of a partially overlapping set of ISGs.6
OVERVIEW OF MODULATION OF INTERFERON RESPONSE IN IMMUNE EVASION AND PATHOGENESIS BY SARS-COV-1, MERS-COV, AND HIV-1
SARS-CoV-1 and Middle East respiratory syndrome coronavirus (MERS-CoV) antagonize IFN production, inhibit IFN signaling, and enhance IFN resistance.23, 24 and 25 SARS-CoV-1 inhibits multiple arms of the IFN-I response, resulting in strongly dampened IFN-β production during infection. The SARS-CoV-1 ORF3b, ORF6, NSP1, NSP3 (papain-like protease), NSP7, NSP15, membrane (M), and nucleocapsid (N) proteins antagonize the IFN response.26, 27, 28, 29, 30 and 31 Mutant SARS-CoV-1 with a bat CoV NSP3 substitution or ORF6 deletion had attenuated IFN antagonism.30,32
The SARS-CoV-1 N protein inhibits recognition by the host cell of SARS-CoV-1 RNA by inhibiting TRIM25 activation of RIG-I and IRF3 phosphorylation. NSP1, NSP3 (papain-like protease), and ORF3b also inhibit IRF3 phosphorylation; and ORF3b and N further inhibit IRF3 translocation to the nucleus. NSP1 additionally targets IRF7 and c-Jun phosphorylation. The membrane (M) protein inhibits assembly of the Traf6 complex, thereby reducing nuclear factor kappa B (NF-κB) import into the nucleus.
Together, these activities result in reduced IFN-β production. NSP1 inhibits STAT1 phosphorylation, and ORF6 inhibits STAT1 translocation to the nucleus, further dampening ISG production.
Together, these activities result in reduced IFN-β production. NSP1 inhibits STAT1 phosphorylation, and ORF6 inhibits STAT1 translocation to the nucleus, further dampening ISG production.
Along with a weak IFN response, an exacerbated immune response has been associated with SARS-CoV-1 and MERS-CoV.33, 34 and 35 In fact, dysregulation of the innate immune response contributes to pathogenicity of the SARS caused by SARS-CoV-1 and SARS-CoV-2, and the severe MERS caused by MERS-CoV.15,36, 37 and 38
In SARS-CoV-1-infected mice, a delayed IFN-I response is associated with high mortality secondary to rapid virus replication, the recruitment of inflammatory monocyte-macrophages to the lungs, and abnormally heightened cytokine and chemokine responses. The latter effects can be prevented by treating mice with IFN-I prior to the peak of virus replication or by depleting inflammatory monocyte-macrophages.15 Similarly, administration of IFN-I to mice prior to infection with MERS-CoV protected from infection, whereas delaying IFN-I treatment until 2 days postinfection resulted in a heightened inflammatory state characterized by recruitment of inflammatory monocyte-macrophages and neutrophils to the lungs and an elevated inflammatory cytokine expression.38 IFN-I treatment was effective only if administered early during MERS-CoV infection.36 Likewise, in patients infected with SARS-CoVs, a prolonged IFN response resulted in a delayed adaptive immune response, the continued upregulation of inflammatory chemokines, and distinct interferon-stimulated genes expression, indicating that IFN production needs to be resolved to initiate and generate a protective adaptive immune response.37
A collection of potent cytokines involved in controlling virus infections and priming adaptive immune responses are generated after CoV infection.39 Indeed, virus pathogenesis is frequently associated with an exacerbated induction of proinflammatory cytokines that is mainly driven by the activation of at least one of the following five pathways: IRF-3 and -7, activating transcription factor (ATF)2/jun, jun/fos (AP-1), NF-κB, and NF-AT. Among them, the NF-κB pathway is the most frequently activated.40,41 NF-κB is a heterogeneous collection of dimers, composed of various combinations of members of the Rel family, which in eukaryotes include p50 (NF-κB1), p52 (NF-κB2), Rel (c-Rel), p65 (RelA), and RelB.
In human immunodeficiency virus (HIV)-1-infected individuals on combination antiretroviral therapy, low levels of IFN-I are thought to be a driving force for immune activation and T-cell exhaustion. Rev-CRM1-dependent nuclear export and peripheral membrane association of intron-containing HIV-1 RNA, independent of primary viral sequence or viral protein expression, are subject to sensing and signaling via MAVS, resulting in IFN-I-dependent proinflammatory responses in macrophages.42 Additionally, HIV-1 intron–containing RNA induces innate immune activation of macrophages, which leads to upregulation of inhibitory receptor expression and functional immune exhaustion of cocultured T cells. These findings suggest that persistent expression of HIV-1 intron–containing RNA in macrophages contributes to chronic immune activation and T-cell dysfunction and that use of HIV RNA expression inhibitors as adjunct therapy might abrogate aberrant inflammation and restore immune function in HIV-infected individuals on combination antiretroviral therapy.42 Whether a similar mechanism operates in SARS-CoV-2 and underlies acute and long-term sequelae in long COVID remains to be determined.
OVERVIEW OF MODULATION OF INTERFERON RESPONSE AND OTHER HOST DEFENSES IN IMMUNE EVASION AND PATHOGENESIS BY SARS-COV-2
As is the case for SARS-CoV-1 and MERS-CoV, infection with SARS-CoV-2 induces an overt but delayed IFN-I response.43 Some patients with severe COVID-19 present with delayed or no induction of IFN-I and -III.44 A unique pathogenic feature of COVID-19 is a heightened suppression of innate immunity and elevated inflammatory responses.45 During the acute infection phase, SARS-CoV-2 inhibits STAT1, which is compensated for by hyperactivation of STAT3 resulting in hypercytokinemia.46, 47 and 48
In the absence of effective IFN expression, SARS-CoV-2 is able to replicate to higher titers, resulting in an exaggerated inflammatory response.49 Conversely, IFN treatment inhibits SARS-CoV-2 infection in a dose-dependent manner in human Calu-3 cells and simian Vero E6 cells.50 The blockade of IFN signaling by SARS-CoV-2 is largely impeded by IFN pretreatment (6 hours preinfection),
whereas postinfection treatment at 16 hours yields only modest results.51 Early administration of pegylated IFN-λ decreases SARS-CoV-2 viral load in outpatients with COVID-19 overall or with uncomplicated disease,52,53 as well as the incidence of hospitalization or an emergency room visit in predominantly vaccinated outpatients with COVID-19.54 IFN-λ, a dominant IFN at the epithelial surface, is more promising for clinical use than type I IFNs because it is less proinflammatory, and pegylated IFN-λ has less off-target effects than pegylated IFN-α/β.
whereas postinfection treatment at 16 hours yields only modest results.51 Early administration of pegylated IFN-λ decreases SARS-CoV-2 viral load in outpatients with COVID-19 overall or with uncomplicated disease,52,53 as well as the incidence of hospitalization or an emergency room visit in predominantly vaccinated outpatients with COVID-19.54 IFN-λ, a dominant IFN at the epithelial surface, is more promising for clinical use than type I IFNs because it is less proinflammatory, and pegylated IFN-λ has less off-target effects than pegylated IFN-α/β.
The fact that the IFN innate immune signaling system in healthy children is primed and ready in a preactivated state across several epithelial cell types may also explain milder COVID-19 disease severity in children compared to adults as a result of increased timely responsiveness to viral attack.55, 56, 57 and 58 Conversely, a study of 1,261 unvaccinated deceased patients and 34,159 individuals of the general population sampled before the COVID-19 pandemic59 documented that autoimmunity to type I IFNs is a strong and common predictor of COVID-19 death. Autoantibodies against type I IFNs increased infection fatality rates and were associated with high relative risk of death, especially when neutralizing both IFN-α2 and IFN-ω. Infection fatality rates increased with age, ranging from approximately 0.17% for individuals younger than 40 years to 26.7% for those aged 80 or older for autoantibodies neutralizing IFN-α2 or IFN-ω, and from 0.84% to 40.5% for autoantibodies neutralizing both.59
The effects of SARS-CoV-2 on the IFN response might be a source of biomarkers for inclusion in scalable point-of-care tests for SARS-CoV-2 that could facilitate early case detection and contact investigation. To this end, among four other genes involved in type I IFN responses, a single blood transcript for IFI27 provided the highest accuracy for discriminating between test-negative controls and test-positive individuals at the time of their first positive SARS-CoV-2 polymerase chain reaction (PCR) result, with high sensitivity and specificity. The transcript performed equally well in individuals with and without symptoms.60
The effects of the IFN response involve various intracellular organelles, and although viruses are simple in structure and composition, their interactions with host cells are complex. Merely to gain entry, animal viruses make use of a repertoire of cellular processes that involve hundreds of cellular proteins. Although viruses such as SARS-CoV-2 by using its spike protein can penetrate into the cytosol directly through the plasma membrane, most, including SARS-CoV-2, can enter the cell via endocytic uptake, vesicular transport through the cytoplasm, and delivery to endosomes and other intracellular organelles. By hijacking a dynamic process through which cells sample their extracellular environment, entry via the endosomal network can also grant SARS-CoV-2 additional protection from innate immunity attack. The internalization may involve clathrin-mediated endocytosis (CME), macropinocytosis, caveolae/lipid raft–mediated endocytosis, or a variety of other still poorly characterized mechanisms. Once internalized, the virus and/or extracellular materials are delivered to a series of membranous organelles within eukaryotes.61
Among intracellular organelles, mitochondria play a role in the IFN response and are at the intersection of key pathways regulating antiviral signaling, the proinflammatory response, and cell death.62 As mentioned earlier, the outer mitochondrial membrane protein MAVS is a critical determinant of antiviral signaling.63,64 After viral infection, viral nucleic acids activate MAVS-dependent antiviral innate immune responses. SARS-CoV-2 infection impairs MAVS-mediated antiviral signaling by inducing autophagy-dependent degradation of MAVS (see ORF10 section) and interacting with components of mitochondrial permeability transition pore (mPTP) to induce autophagy and mitochondrial clearance.65, 66 and 67 The SARS-CoV-2 ORF9b and NSP4 proteins cause extensive mitochondrial structural changes, outer membrane macropore formation, and the release of inner membrane vesicles loaded with mitochondrial DNA (see ORF9b and NSP4 sections).68 SARS-CoV-2 infection also impairs mitophagy.69 Mitochondria are therefore essential for SARS-CoV-2 replication and could be used for therapy, as exemplified by mesenchymal stem cell (MSC)-mediated delivery of engineered macropore-deficient mitochondria to SARS-CoV-2-infected cells.68
SARS-COV-2 PROTEINS IN IMMUNE EVASION AND PATHOGENESIS
As summarized in Table 7.1,7.2 to 7.3 and detailed by protein, most SARS-CoV-2 proteins antagonize the IFN response43,70 and other forms of innate and also adaptive immunity. The collective effect from all modulating proteins determines the final antagonism for each virus.25 Different levels of viral
replication and immune antagonism dictate viral transmission and disease development. For instance, when infecting human lung tissues, SARS-CoV-2 produces significantly more virus but less IFN and proinflammatory cytokines/chemokines than SARS-CoV-1, providing an explanation for more asymptomatic transmission and delayed disease onset of COVID-19.71 CoVs also generate microRNAs that relate to host-pathogen interaction and viral pathogenicity (see “SARS-CoV-2 MicroRNAs and Immune Evasion and Pathogenesis” section).72
replication and immune antagonism dictate viral transmission and disease development. For instance, when infecting human lung tissues, SARS-CoV-2 produces significantly more virus but less IFN and proinflammatory cytokines/chemokines than SARS-CoV-1, providing an explanation for more asymptomatic transmission and delayed disease onset of COVID-19.71 CoVs also generate microRNAs that relate to host-pathogen interaction and viral pathogenicity (see “SARS-CoV-2 MicroRNAs and Immune Evasion and Pathogenesis” section).72
Accessory Proteins (Open Reading Frames 3a, 3b, 6, 7a, 7b, 8, 9b, 9c, 10)
TABLE 7.1 Modulation of host defenses and pathogenic pathways by SARS-CoV-2 proteins: Accessory Proteins | ||||||||||||||||||||||||||||||||||||||||||||||||||||||||||||||||||||||||||||||||||||||||||||||||||||||||||||||||||||||||||||||||||||||||||||||||||||||||||||||||||||||||||||||||||||||||||||||||||||||||||||||||||
---|---|---|---|---|---|---|---|---|---|---|---|---|---|---|---|---|---|---|---|---|---|---|---|---|---|---|---|---|---|---|---|---|---|---|---|---|---|---|---|---|---|---|---|---|---|---|---|---|---|---|---|---|---|---|---|---|---|---|---|---|---|---|---|---|---|---|---|---|---|---|---|---|---|---|---|---|---|---|---|---|---|---|---|---|---|---|---|---|---|---|---|---|---|---|---|---|---|---|---|---|---|---|---|---|---|---|---|---|---|---|---|---|---|---|---|---|---|---|---|---|---|---|---|---|---|---|---|---|---|---|---|---|---|---|---|---|---|---|---|---|---|---|---|---|---|---|---|---|---|---|---|---|---|---|---|---|---|---|---|---|---|---|---|---|---|---|---|---|---|---|---|---|---|---|---|---|---|---|---|---|---|---|---|---|---|---|---|---|---|---|---|---|---|---|---|---|---|---|---|---|---|---|---|---|---|---|---|---|---|---|
|
A unique characteristic of CoVs is the inclusion of genes in the 3´ end of their genome. Each member of the CoV family has a distinct set of these so-called accessory genes because they are not essential for replication.73,74 Many of these CoV genes alter host cell pathways including IFN signaling, cycle progression, and various antiviral responses to viral infection with multifaceted effects on viral replication and pathogenesis.32,75, 76 and 77
ORF3a
ORF3a is a virus-encoded ion channel or viroporin present in SARS-CoV-2 and SARS-CoV-1 but not in the less pathogenic common cold causing hCOV-HKU1 and -OC43. ORF3a suppresses the innate immune response via various mechanisms including disrupting the host ubiquitin system and inhibiting stimulator of IFN gene (STING), which induce IFN-I production and NF-κB signaling.78,79
One mechanism whereby ORF3a suppresses innate immunity is by affecting protein ubiquitination, a dynamic multifaceted posttranslational modification involved in nearly all aspects of eukaryotic biology, including viral infection.80 This modification plays a key role in modulating the immune response,81 and viruses, such as yellow fever, have evolved to evade immune responses by subverting the host ubiquitin system.82,83 Once attached to a substrate, the 76-amino-acid protein ubiquitin is subjected to further modifications, creating a multitude of distinct signals with distinct cellular outcomes, referred to as the “ubiquitin code.”
Ubiquitin can be ubiquitinated on seven lysine residues or on the amino-terminus, leading to polyubiquitin chains that can encompass complex topologies. Alternatively, or in addition, ubiquitin lysine residues can be modified by ubiquitin-like molecules (such as SUMO or NEDD8). Finally, ubiquitin can also be acetylated on lysine, or phosphorylated on serine, threonine, or tyrosine residues, and each modification has the potential to dramatically alter the signaling outcome.
Although there is a plethora of distinctly modified ubiquitin species in cells, much progress has been made to characterize the roles of distinct ubiquitin modifications, and many enzymes and receptors have been identified that create, recognize, or remove these ubiquitin modifications.77 SARS-CoV-2 ORF3a usurps the E3 ubiquitin ligase TRIM59,84 which regulates antiviral innate immune signaling.85 Among SARS-CoV-2 proteins other than ORF3a with IFN-I antagonism,79 NSP13 and ORF7a also seem to be ubiquitinated.86 Future studies are needed to characterize the molecular details and biologic functions of this ubiquitination.
Host innate immune responses are potent barriers to the cross-species transmission of viruses,87, 88 and 89 and RNA viruses overall trigger and are inhibited by cGAS-STING activation.90 ORF3a, as does NSP5, binds to diverse vertebrate STINGs, including those from humans, mice, and chickens, and inhibits cGAS-STING, thereby blocking the nuclear accumulation of p65 to inhibit NF-κB signaling but not the RLR response.78
Binding of STINGs from different species is consistent with the ability of SARS-CoV-2 to infect a wide range of animals.78 Bats are believed to be a natural host for many CoVs,87,91 and STING molecules in all the bats examined to date are defective for the induction of type I IFN and have compromised antiviral activity.92 This defective STING activity has been linked to the persistence of CoV infection in bats. Apparently, SARS-CoV-2 has maintained or regained the ability to inhibit STING function during cross-species transmission.78
ORF3b
SARS-CoV-2 ORF3b is a potent antagonist of IFN signaling, suppressing the induction of type I IFN more efficiently than its SARS-CoV-1 ortholog.93 One of the features distinguishing SARS-CoV-2 from SARS-CoV-1 is the presence of premature stop codons in its ORF3b gene. Phylogenetic analyses and functional assays reveal that SARS-CoV-2-related viruses from bats and pangolins also encode truncated ORF3b gene products with strong anti-IFN activity. Natural SARS-CoV-2 quasispecies with an extended ORF3b gene have emerged that may potentially affect COVID-19 pathogenesis.93 ORF3b or N proteins do not inhibit nuclear translocation but phosphorylation of STAT1.29
ORF6
The SARS-CoV-1 and SARS-CoV-2 ORF6 proteins localize to the endoplasmic reticulum/Golgi membrane and nuclear pore complex in infected cells, where it binds to and disrupts nuclear import
complex formation by tethering the importin karyopherin alpha 2 (KPNA2) and karyopherin beta 1 to the membrane, thereby inhibiting IRF3 nuclear translocation.79 SARS-CoV-1 and SARS-CoV-2 ORF6 proteins have been shown to directly interact with the mRNA export factor nuclear pore protein (Nup)98 and Rae1 via its C-terminal domain (CTD) to impair docking of cargo-receptor (karyopherin/importin) complex and disrupt nuclear import.94, 95 and 96 A methionine-to-arginine substitution at residue 58 impairs ORF6 binding to the Nup98-Rae1 complex and abolishes its IFN antagonistic function.43,94 ORF6 inhibits the export of many mRNAs including those encoding antiviral factors such as IRF1 and RIG-I.96,97 Retention of import factors at the endoplasmic reticulum/Golgi membrane leads to a loss of nuclear translocation of STAT1 in response to IFN signaling, thus blocking the expression of STAT1-activated genes that establish an antiviral state.32 SARS-CoV-2 ORF6 also inhibits the RLR pathway.43,70
complex formation by tethering the importin karyopherin alpha 2 (KPNA2) and karyopherin beta 1 to the membrane, thereby inhibiting IRF3 nuclear translocation.79 SARS-CoV-1 and SARS-CoV-2 ORF6 proteins have been shown to directly interact with the mRNA export factor nuclear pore protein (Nup)98 and Rae1 via its C-terminal domain (CTD) to impair docking of cargo-receptor (karyopherin/importin) complex and disrupt nuclear import.94, 95 and 96 A methionine-to-arginine substitution at residue 58 impairs ORF6 binding to the Nup98-Rae1 complex and abolishes its IFN antagonistic function.43,94 ORF6 inhibits the export of many mRNAs including those encoding antiviral factors such as IRF1 and RIG-I.96,97 Retention of import factors at the endoplasmic reticulum/Golgi membrane leads to a loss of nuclear translocation of STAT1 in response to IFN signaling, thus blocking the expression of STAT1-activated genes that establish an antiviral state.32 SARS-CoV-2 ORF6 also inhibits the RLR pathway.43,70
ORF7a
The ORF7a proteins of SARS-CoV-1 and -2 are type I transmembrane proteins with an immunoglobulin (Ig)-like fold (PF08779),97,98 known for its structural and functional versatility.99 ORF7a is expressed and retained intracellularly, and its short cytoplasmic tail and transmembrane domain have been implicated in trafficking of the protein within the endoplasmic reticulum and Golgi network.97 ORF7a acts as an immune antagonist of the host cell by interfering with the ISG BST2.76,86
SARS-CoV-2 usurps the host ubiquitin system to polyubiquitinate ORF7a at lysine 119. This polyubiquitination is primarily formed by K63-linked ubiquitin chains and enhances ORF7a to inhibit type I IFN signaling via STAT2 phosphorylation.87
SARS-CoV-2 ORF7a activates NF-κB activation through associations with TAK1. K63-linked polyubiquitination of ORF7a by RNF121 appears essential for NF-κB activation.100 ORF7a protein induces the NF-κB-dictated proinflammatory cytokines including interleukin (IL)-1α, IL-1β, IL-6, IL-8, IL-10, tumor necrosis factor (TNF)-α, and IFN-β.101 ORF7a also induces IL-3, IL-4, IL-7, and IL-23. Of 15 chemokines examined, CCL11, CCL17, CCL19, CCL20, CCL21, CCL22, CCL25, CCL26, CCL27, and CXCL9 are significantly upregulated by ORF7. These cytokines and chemokines are frequently elevated in severely ill patients with COVID-19. ORF7a may therefore be a therapeutic target to minimize uncontrolled inflammation in patients with COVID-19.100,101
ORF7b
ORF8
As other accessory proteins, the ORF8 proteins of SARS-CoVs do not participate in viral replication,108,109 but can affect viral pathogenesis.98 Probably driven by the race between virus and host, ORF8 is an evolutionary hot spot,110 one of the most rapidly evolving segments of the SARS-CoV-2 genome,98 and thus ORF8 is the least conserved protein of SARS-CoV-2.111,112
SARS-CoV-1 encoded a single protein (ORF8ab) in earlier human and animal isolates113; however, in later human isolates during the peak of the 2003 SARS-CoV-1 epidemic, a 29-nucleotide deletion in the center of the gene split ORF8 into two smaller ORFs, encoding proteins ORF8a and ORF8b.114,115 The ORF8a polypeptide (39 amino acids), a viroporin, of SARS-CoV-1 is present in mitochondria, and its overexpression results in increased mitochondrial transmembrane potential, production of reactive oxygen species, increased caspase 3 activity, and cellular apoptosis.116,117 When reconstituted into artificial lipid bilayers, ORF8a forms cation-selective ion channels, and computational modeling studies predicted a putative homo-oligomeric helical bundle model.118 In SARS-CoV-1, full-length E and ORF3a viroproteins are required for maximal virus replication and virulence, whereas viroporin ORF8a has only a minor impact on virus viability.119
The 84-amino-acid-long SARS-CoV-1 ORF8b protein induces the activation of the transcription factor ATF6 to facilitate protein folding and processing120,121 and triggers intracellular stress
by activating the nucleotide-binding oligomerization domain–like receptor family pyrin domain–containing 3 (NLRP3) inflammasome,122 whereas the 39-amino-acid-long ORF8a protein induces cellular apoptosis. The ORF8a/b protein pair suppresses the IFN signaling pathway, and ORF8b can also mediate this process via ubiquitin-proteasome-dependent degradation of IRF3.123 Moreover, ORF8b downregulates the expression of the viral E protein, but not in concert as ORF8ab does.124 Notably, ORF8a interacts with S; ORF8b interacts with M, E, ORF3a, and ORF7a; and ORF8ab interacts with S, ORF3a, ORF7a,124 and to a lesser extent with M.124,125 The split structure of ORF8a and ORF8b appears to facilitate a more efficient replication against IFN, thereby increasing virulence.121,122 Because ORF8b downregulates protein E, which in turn is known to have a positive effect on virus growth, it has been suggested that ORF8b might play a crucial role in modulating virulence.125
by activating the nucleotide-binding oligomerization domain–like receptor family pyrin domain–containing 3 (NLRP3) inflammasome,122 whereas the 39-amino-acid-long ORF8a protein induces cellular apoptosis. The ORF8a/b protein pair suppresses the IFN signaling pathway, and ORF8b can also mediate this process via ubiquitin-proteasome-dependent degradation of IRF3.123 Moreover, ORF8b downregulates the expression of the viral E protein, but not in concert as ORF8ab does.124 Notably, ORF8a interacts with S; ORF8b interacts with M, E, ORF3a, and ORF7a; and ORF8ab interacts with S, ORF3a, ORF7a,124 and to a lesser extent with M.124,125 The split structure of ORF8a and ORF8b appears to facilitate a more efficient replication against IFN, thereby increasing virulence.121,122 Because ORF8b downregulates protein E, which in turn is known to have a positive effect on virus growth, it has been suggested that ORF8b might play a crucial role in modulating virulence.125
During the pandemic, SARS-CoV-2 had expressed ORF8ab instead of ORF8a and ORF8b. ORF8ab, which is absent in the more lethal MERS-CoV,126 does not have a structure that is compatible with an ion channel. The intact ORF8 contains a cleavable signal sequence, directing the precursor to the endoplasmic reticulum and mediating its translocation into the lumen. The cleaved protein is N-glycosylated and remains stable in the endoplasmic reticulum.115 ORF8 activates IL-17 signaling pathway and promotes the expression of proinflammatory factors, thereby contributing to the observed cytokine storm in COVID-19,127 independent of viroporin activity. SARS-CoV-2 ORF8 dysregulates the tumor growth factor (TGF)-β pathway, known for its involvement in tissue fibrosis.128 The structure of ORF8 adopts an Ig-like fold and forms a disulfide-linked homodimer containing an intermolecular parallel β-sheet.129
Crystal structure of ORF8 of SARS-CoV-2 revealed a 60-amino-acid core like that of SARS-CoV-2 ORF7a, from which ORF8 has been postulated to originate by nonhomologous recombination.130 Unique to SARS-CoV-2 ORF8 are two dimerization interfaces, one covalent and the other noncovalent.129 In the carboxyl-terminus of ORF8, arginine 115, aspartic acid 119, phenylalanine 120, and isoleucine 121 contribute to the covalent dimer interface, with arginine 115 and aspartic acid 119 forming salt bridges that flank a central hydrophobic core in which valine 117 interacts with its symmetry-related counterpart.129
ORF8 as a glycoprotein homodimer interacts with intracellular transport signaling, leading to downregulation of MHC-I by selective targeting for lysosomal degradation via autophagy131 and/or extracellular signaling involving IFN-I signaling,132 mitogen-activated protein kinase (MAPK) growth pathways,133 the TGF-β1 signaling cascade,128 and IL-17 signaling promoting inflammation and contributing to the COVID-19-associated cytokine storm.127 SARS-CoV-2 ORF8 induces endoplasmic reticulum stress by triggering the ATF6 and inositol-requiring enzyme 1 (IRE1) branches of the endoplasmic reticulum stress pathway, but not the PERK pathway, and functions as an IFN antagonist to inhibit the production of IFN-β and ISG15 and ISG56 induced by polyinosinic-polycytidylic acid (poly (I:C)) and decreasing nuclear translocation of IRF3.134 SARS-CoV-2 ORF8 antagonizes the RIG-I/MDA-5 signaling pathway by targeting HSP90B1, which subsequently exhibits an inhibitory effect on the production of IFN-I.135 Deletion of ORF8 decreased severity of SARS-CoV-2 infection in a cohort study. However, these functions for ORF8 have been debated.136
As mentioned earlier, the replication machinery of SARS-CoV-2 is closely associated with the endoplasmic reticulum in host cells and SARS-CoV-2 hijacks the activation of the unfolded protein response (UPR) to facilitate its replication and suppress host innate immunity. Although SARS-CoV-2 ORF8 lacks a conventional carboxyl-terminal KDEL motif for endoplasmic reticulum retention, an interaction landscape study revealed that ORF8 was at the top of SARS-CoV-2 proteins interacting with the early secretory pathway in host cells.84 In fact, ORF8 interacting proteins are enriched in the endoplasmic reticulum protein quality control system including protein folding, glycosylation, and endoplasmic reticulum–associated degradation (ERAD).137 SARS-CoV-2 ORF8 protein accumulates in the endoplasmic reticulum and escapes the degradation system by forming mixed disulfide complexes with endoplasmic reticulum oxidoreductases. ORF8 induces the activation of three UPR pathways through targeting key UPR components, remodels endoplasmic reticulum morphology, and accelerates protein trafficking. Moreover, small-molecule reducing agents release ORF8 from the mixed disulfide complexes and facilitate its degradation, therefore mitigating endoplasmic reticulum stress. Via this unique mechanism, SARS-CoV-2 ORF8 escapes degradation by host cells and regulates endoplasmic reticulum reshaping. Targeting ORF8-involved mixed
disulfide complexes could be a new strategy to alleviate SARS-CoV-2-induced endoplasmic reticulum stress and related diseases.137
disulfide complexes could be a new strategy to alleviate SARS-CoV-2-induced endoplasmic reticulum stress and related diseases.137
Approximately 5% of CD4+ T cells in most COVID-19 cases are specific for ORF8, and ORF8 accounts for 10% of CD8+ T-cell reactivity in COVID-19 recovered subjects.138,139 Moreover, anti-ORF8 antibodies have been detected in both symptomatic and asymptomatic patients early during infection by SARS-CoV-2,140 and some diagnostic assays for SARS-CoV-2 infection target accessory genes or proteins such as ORF8.141
Another effect of SARS-CoV-2 ORF8 involves epigenetic mechanisms that affect COVID-19 outcomes by regulating IFN signaling, angiotensin-converting enzyme 2 (ACE2), and immunity-related genes that particularly escape from X chromosome inactivation.142 The role of ORF8 in chromatin disruption early in infection adds to the list of proposed roles covered here and affecting other cellular compartments or at later stages of infection.127,131,134,135,143
Beyond suppressing the innate antiviral host response, SARS-CoV-2 infection disrupts epigenetic regulation as suggested by topoisomerase inhibition protecting against SARS-CoV-2-induced lethal inflammation144, 145, 146 and 147; virus-induced senescence-associated secretory phenotype comprising proinflammatory cytokines, extracellular matrix–active factors and procoagulation mediators148; and non-cell-autonomous-driven anosmia preceded by a dramatic reorganization of the neuronal nuclear architecture, which results in dissipation of genomic compartments harboring olfactory receptor genes.149
The SARS-CoV-2 but not the SARS-CoV-1 ORF8 gene contains an alanine-arginine-lysine-serine (ARKS) motif, which is found at two distinct sites in the histone H3 tail, through which ORF8 expression disrupts regulation of histone posttranslational modification.150 ORF8 is associated with chromatin-associated proteins, histones, and the nuclear lamina and is itself acetylated within the histone mimic ARKS motif. ORF8 expression disrupts multiple critical histone posttranslational modifications and promotes chromatin compaction, whereas ORF8 lacking the histone mimic motif does not. Furthermore, SARS-CoV-2 infection in human cell lines and postmortem patient lung tissue causes similar global disruptions to chromatin acting partly through the histone mimic. In addition, deletion of the ORF8 gene or the sequence encoding the histone mimic affects the host cell transcriptional response to SARS-CoV-2 infection. Finally, loss of ORF8 decreases the replication of SARS-CoV-2 in human-induced pluripotent stem cell–derived iAT2 pulmonary cells, whereas loss of the histone mimic motif specifically affects viral genome copy number.150
ORF9b
ORF9b immediately accumulates and antagonizes the antiviral type I IFN response during SARS-CoV-2 infection on primary human pulmonary alveolar epithelial cells. ORF9b targets the NF-κB essential modulator (NEMO) and interrupts its K63-linked polyubiquitination upon viral stimulation, thereby inhibiting the canonical IKKα/β/γ-NF-κB signaling and subsequent IFN production.151
ORF9b interacts with TOM70,86,152 a surface receptor of the translocase of the outer membrane TOM complex that is the gateway of protein import in mitochondria. TOM70 acts as an essential versatile adaptor linking MAVS to TBK1/IRF3, resulting in the activation of IRF3.153 The crystal structure of SARS-CoV-2 ORF9b in complex with the cytosolic segment of human TOM70 revealed that a central portion of ORF9b occupies the deep pocket in the TOM70 CTD and adopts a helical conformation different from the β-sheet-rich structure of the ORF9b homodimer.152 Interactions between ORF9b and TOM70 CTD are primarily hydrophobic and endothermic in contrast to the exothermic electrostatic interaction between the heat shock protein 90 (Hsp90) C-terminal EEVD motif and the TOM70 N-terminal clamp domain (R192) that recruits TBK1/IRF3 to mitochondria. Disruption of the latter interaction or altered localization of TOM70 sharply impairs activation of TBK1 and IRF3.153 In fact, the binding affinity of Hsp90 EEVD motif to TOM70 N-terminal domain (NTD) is reduced by approximately 29-fold when ORF9b occupies the pocket of TOM70 CTD, supporting the hypothesis that ORF9b allosterically inhibits the Hsp90/TOM70 interaction,152 thus ultimately weakening the signaling cascade for IFN activation.153,154
SARS-CoV-2 infection is associated with extensive mitochondrial damage,155 high extracellular mitochondrial DNA levels,156 and impaired antiviral signaling.145 Damaged mitochondria promote the release of mitochondrial DNA into the cytosol or the extracellular space. In the cytosol,
mitochondrial DNA activates the classical cGAS/STING1-mediated IFN signaling and NLRP3 inflammasome formation.157 Extracellular mitochondrial DNA is a potential driver of the proinflammatory response.158, 159, 160 and 161 In particular, SARS-CoV-2 ORF9b, as does NSP4 (see NSP4 section), causes extensive mitochondrial structural changes, outer membrane macropore formation, and the release of inner membrane vesicles loaded with mitochondrial DNA. ORF9b inhibits the antiapoptotic member of the B-cell lymphoma 2 (BCL2) family protein myeloid cell leukemia-1 (MCL1) and induces inner membrane vesicle formation containing mitochondrial DNA, whereas the macropore-forming ability of NSP4 is mediated via interaction with BCL2 antagonist/killer (BAK) (see more details on mitochondrial effects of ORF9b under NSP4 section).69
mitochondrial DNA activates the classical cGAS/STING1-mediated IFN signaling and NLRP3 inflammasome formation.157 Extracellular mitochondrial DNA is a potential driver of the proinflammatory response.158, 159, 160 and 161 In particular, SARS-CoV-2 ORF9b, as does NSP4 (see NSP4 section), causes extensive mitochondrial structural changes, outer membrane macropore formation, and the release of inner membrane vesicles loaded with mitochondrial DNA. ORF9b inhibits the antiapoptotic member of the B-cell lymphoma 2 (BCL2) family protein myeloid cell leukemia-1 (MCL1) and induces inner membrane vesicle formation containing mitochondrial DNA, whereas the macropore-forming ability of NSP4 is mediated via interaction with BCL2 antagonist/killer (BAK) (see more details on mitochondrial effects of ORF9b under NSP4 section).69
ORF9c
The SARS-CoV-2 ORF9c is involved in viral immune evasion via interaction with multiple proteins that modulate IKK and NF-kB signaling pathway,86 including NLRX1, F2RL1, and NDFIP2.162, 163 and 164 In human pluripotent stem cell–derived cardiomyocytes, ORF9c overexpression significantly reduced cellular adenosine triphosphate (ATP) level, induced apoptosis, and caused electrical dysfunctions.165
ORF10
Compared with the SARS-CoV-1 genome, that of SARS-CoV-2 uniquely has the potential to encode the 38-amino-acid ORF10 protein,166,167 which contains multiple cytotoxic T-lymphocyte epitopes168 and has been associated, mainly cell studies, with immune evasion via mitophagy-mediated degradation of MAVS and with pathogenesis via ubiquitination and degradation of host cell proteins. The debate on whether ORF10 is part of the SARS-CoV-2 transcriptome beckons resolution.
In the immune system, proper mitochondrial function is a prerequisite for inflammatory responses and host defense.169 Some viral infections affect the host mitochondrial network by inducing and controlling mitophagy through different strategies, which allow them to promote persistent infection and attenuate innate immune responses.170 For example, the matrix protein of human parainfluenza virus 3 induces Parkin-PINK1-independent complete mitophagy, inhibiting host antiviral IFN responses,171 and human herpesvirus 8–encoded viral IRF 1 (vIRF-1) promotes mitochondrial clearance by binding to a mitophagy receptor, the Nip3-like protein X (NIX), on mitochondria and activating NIX-mediated mitophagy to support viral replication.172 NIX is a typical autophagy receptor that is able to transport mitochondria to autophagosomes through direct interaction with Atg8 family proteins.173 NIX-mediated mitophagy is responsible for the elimination of spontaneously aggregated MAVS.174 Therefore, successful viral infection and replication are largely achieved by the ability of viruses to attenuate the innate antiviral responses mediated by mitochondria.169
ORF10 induces mitophagy-mediated degradation of MAVS by binding to NIX.65 Overexpression of ORF10 markedly suppressed the expression of IFN-I genes and ISGs by inducing mitophagy with MAVS degradation and LC3 accumulation in mitochondria. Mechanistically, ORF10 is translocated to mitochondria by interacting with the mitophagy receptor NIX and induces mitophagy through its interaction with both NIX and LC3B. Knockdown of NIX expression blocks mitophagy activation, MAVS degradation, and IFN-I signaling pathway inhibition by ORF10. In SARS-CoV-2 infection, ORF10 inhibited MAVS expression and facilitated viral replication.65
In a study using HEK293 cells that generate a poor innate immune response to transfected SARS-CoV-2 ORF10, ORF8, and M genes, treatment with cannabidiol augmented innate antiviral response as manifested by increased expression of IFNγ, IFNλ1, and IFNλ2/3, as well as the OAS 1-2 and L without enhancing apoptosis. Cannabidiol similarly enhanced the cellular antiviral response to poly (I:C), suggesting that it may prime components of the innate immune system, thereby increasing readiness to respond to RNA-type viral infection without activating apoptosis, and could be studied for potential use as prophylaxis.175
In terms of pathogenesis of COVID-19, ORF10 binds to an E3 ubiquitin ligase complex containing Cullin-2, Rbx1, Elongin B, Elongin C, and ZYG11B, which ubiquitinates host proteins and degrades them via the 26 S proteasome; however, ZYG11B is dispensable for SARS-CoV-2 infection, as is the ORF10-Cullin-2-ZYG11B interaction.176 Nonetheless, SARS-CoV-2 ORF10 by hijacking and enhancing activity of Cullin22-ZYG11B causes increased ubiquitination and subsequent proteasome-mediated degradation of multiple cilium-related protein, including an intraflagellar transport (IFT) complex B protein, IFT46, thereby impairing the delivery of new axonemal
components and both cilia biogenesis and maintenance.177 Exposure of the respiratory tract of ACE2 humanized mice to Spike-lentivirus-ORF10 caused multiple pathogenic phenotypes. The latter observations offer an etiopathologic explanation for how SARS-CoV-2 causes multiple cilia dysfunction–related symptoms specific to COVID-19.177 Numerous odorant receptors accumulate on sensory cilia in the olfactory epithelium, and cilia dysfunction impacts lung diseases and anosmia.178,179
components and both cilia biogenesis and maintenance.177 Exposure of the respiratory tract of ACE2 humanized mice to Spike-lentivirus-ORF10 caused multiple pathogenic phenotypes. The latter observations offer an etiopathologic explanation for how SARS-CoV-2 causes multiple cilia dysfunction–related symptoms specific to COVID-19.177 Numerous odorant receptors accumulate on sensory cilia in the olfactory epithelium, and cilia dysfunction impacts lung diseases and anosmia.178,179
Nonstructural Proteins (1, 2, 3, 4, 5, 6, 9, 10/14/16, 12, 13, and 15)
TABLE 7.2 Modulation of host defenses and pathogenic pathways by SARS-CoV-2 proteins: Nonstructural Proteins | |||||||||||||||||||||||||||||||||||||||||||||||||||||||||||||||||||||||||||||||||||||||||||||||||||||||||||||||||||||||||||||||||||||||||||||||||||||||||||||||||||||||||||||||||||||||||||||||||||||||||||||||||||||||||||||||||||||||||||||||||||||||||||||||||||||||||||||||||
---|---|---|---|---|---|---|---|---|---|---|---|---|---|---|---|---|---|---|---|---|---|---|---|---|---|---|---|---|---|---|---|---|---|---|---|---|---|---|---|---|---|---|---|---|---|---|---|---|---|---|---|---|---|---|---|---|---|---|---|---|---|---|---|---|---|---|---|---|---|---|---|---|---|---|---|---|---|---|---|---|---|---|---|---|---|---|---|---|---|---|---|---|---|---|---|---|---|---|---|---|---|---|---|---|---|---|---|---|---|---|---|---|---|---|---|---|---|---|---|---|---|---|---|---|---|---|---|---|---|---|---|---|---|---|---|---|---|---|---|---|---|---|---|---|---|---|---|---|---|---|---|---|---|---|---|---|---|---|---|---|---|---|---|---|---|---|---|---|---|---|---|---|---|---|---|---|---|---|---|---|---|---|---|---|---|---|---|---|---|---|---|---|---|---|---|---|---|---|---|---|---|---|---|---|---|---|---|---|---|---|---|---|---|---|---|---|---|---|---|---|---|---|---|---|---|---|---|---|---|---|---|---|---|---|---|---|---|---|---|---|---|---|---|---|---|---|---|---|---|---|---|---|---|---|---|---|---|---|---|---|---|---|---|---|---|---|---|---|---|---|---|---|---|
|
NSP1
NSP1 of SARS-CoV-2 is one of the main immune evasion proteins.80,180, 181, 182, 183 and 184 In HEK 293T cells, expression of NSP1 or nucleocapsid in the absence of other viral proteins was sufficient to block IFN induction, but only NSP1 was able to inhibit IFN signaling.51 The NSP1s of MERS-CoV, SARS-CoV-1, and SARS-CoV-2 block IFN signaling by inhibiting host protein translation.28,181,183,185,186 The SARS-CoV-2 NSP1 uses its CTD to block the mRNA entry channel of the 40S ribosome,182,183 thereby inhibiting 80S subunit formation and host protein synthesis.180,184 The NTD and CTD of NSP1 coordinate to drive a tuned ratio of viral to host translation, likely to maintain a certain level of host fitness while maximizing replication. The SL1 region of the SARS-CoV-2 5´-UTR is necessary and sufficient for the virus to evade NSP1-mediated translational suppression.
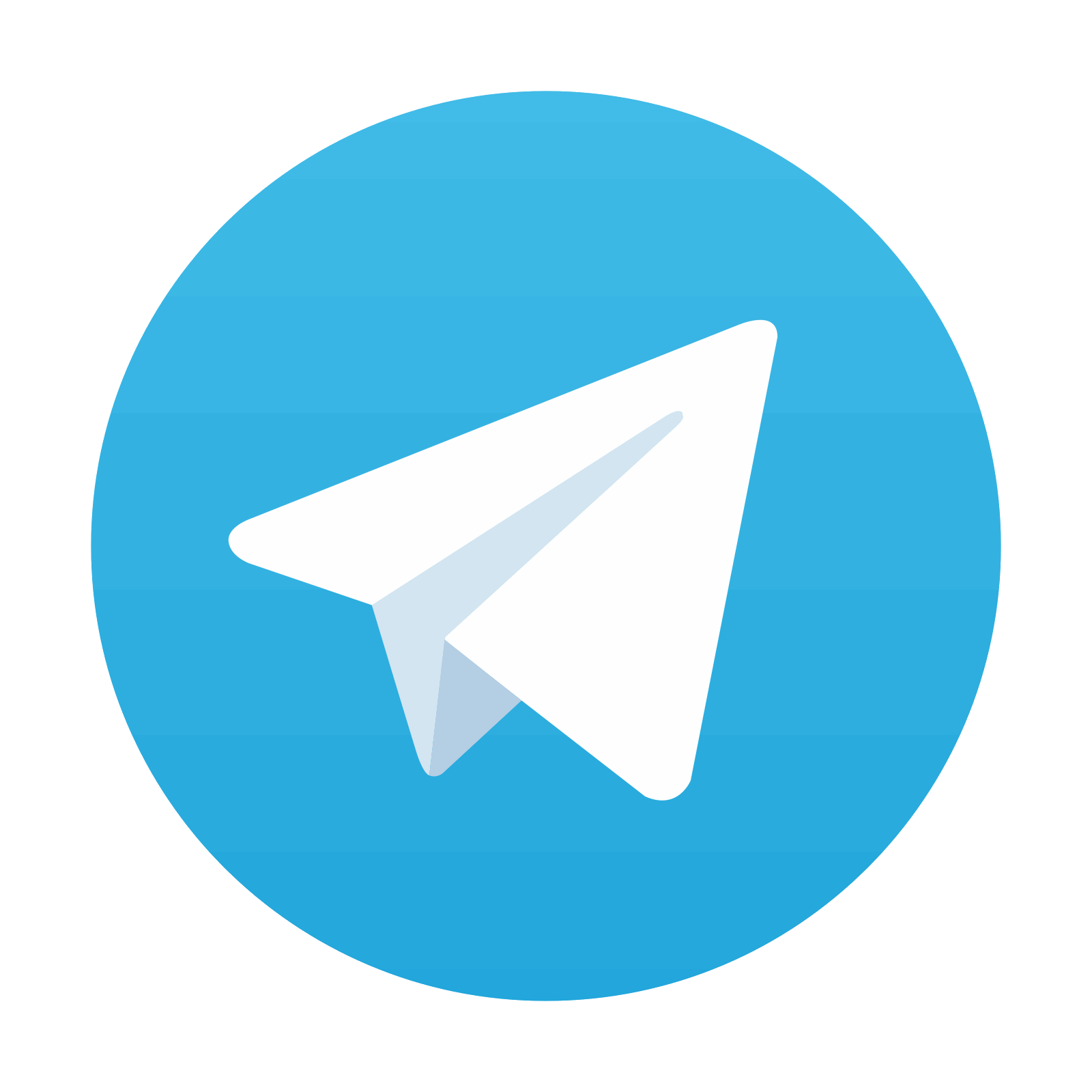
Stay updated, free articles. Join our Telegram channel

Full access? Get Clinical Tree
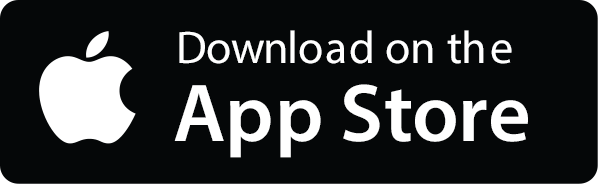
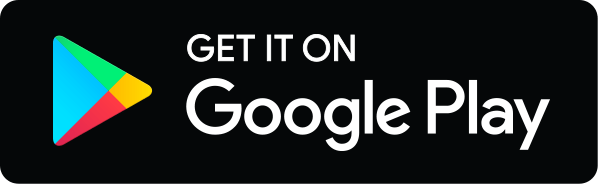
