KEY POINTS
Benign esophageal disease is common and is best evaluated with thorough physiologic testing (high resolution esophageal motility, 24 hour ambulatory pH measurement, and/or esophageal impedance testing) and anatomic testing (esophagoscopy, video esophagography, and/or CT scanning).
GERD is the most common disease of the gastrointestinal tract for which patients seek medical therapy. When GERD symptoms (heartburn, regurgitation, chest pain, and/or supraesophageal symptoms) are troublesome despite adequately dosed PPI, surgical correction may be indicated.
Barrett’s esophagus is the transformation of the distal esophageal epithelium from squamous to a specialized columnar epithelium capable of further neoplastic progression. The detection of Barrett’s esophagus on endoscopy and biopsy increases the future risk of cancer by >40x compared to individuals without Barrett’s esophagus.
Giant hiatal hernia, otherwise known as paraesophageal hernia, should be repaired when symptomatic or associated with iron deficiency anemia. Laparoscopic hiatal hernia repair with fundoplication is the most common approach to repair.
Achalasia is the most common primary esophageal motor disorder. It is characterized by an absence of peristalsis and a hypertensive nonrelaxing lower esophageal sphincter. It is best treated with laparoscopic Heller myotomy and partial fundoplication.
Most esophageal cancer presents with dysphagia, at which time it has invaded the muscularis of the esophagus and is often associated with lymph node metastases. The preferred treatment at this stage is multimodality therapy with chemoradiation therapy followed by open or minimally invasive esophagectomy.
SURGICAL ANATOMY
The esophagus is a muscular tube that starts as the continuation of the pharynx and ends as the cardia of the stomach. When the head is in a normal anatomic position, the transition from pharynx to esophagus occurs at the lower border of the sixth cervical vertebra. Topographically this corresponds to the cricoid cartilage anteriorly and the palpable transverse process of the sixth cervical vertebra laterally (Fig. 25-1). The esophagus is firmly attached at its upper end to the cricoid cartilage and at its lower end to the diaphragm; during swallowing, the proximal points of fixation move craniad the distance of one cervical vertebral body.
Figure 25-1.
A. Topographic relationships of the cervical esophagus: (a) hyoid bone, (b) thyroid cartilage, (c) cricoid cartilage, (d) thyroid gland, (e) sternoclavicular. B. Lateral radio-graphic appearance with landmarks identified as labeled in A. The location of C6 is also included (f). (Reproduced with permission from Rothberg M, DeMeester TR: Surgical anatomy of the esophagus, in Shields TW (ed): General Thoracic Surgery, 3rd ed. Philadelphia: Lea & Febiger, 1989, p 77.)
The esophagus lies in the midline, with a deviation to the left in the lower portion of the neck and upper portion of the thorax, and returns to the midline in the midportion of the thorax near the bifurcation of the trachea (Fig. 25-2). In the lower portion of the thorax, the esophagus again deviates to the left and anteriorly to pass through the diaphragmatic hiatus.
Figure 25-2.
Barium esophagogram. A. Posterior-anterior view. White arrow shows deviation to left. Black arrow shows return to midline. B. Lateral view. Black arrow shows anterior deviation. (Reproduced with permission from Rothberg M, DeMeester TR: Surgical anatomy of the esophagus, in Shields TW (ed): General Thoracic Surgery, 3rd ed. Philadelphia: Lea & Febiger, 1989, p 77.)
Three normal areas of esophageal narrowing are evident on the barium esophagogram or during esophagoscopy. The uppermost narrowing is located at the entrance into the esophagus and is caused by the cricopharyngeal muscle. Its luminal diameter is 1.5 cm, and it is the narrowest point of the esophagus. The middle narrowing is due to an indentation of the anterior and left lateral esophageal wall caused by the crossing of the left main stem bronchus and aortic arch. The luminal diameter at this point is 1.6 cm. The lowermost narrowing is at the hiatus of the diaphragm and is caused by the gastroesophageal sphincter mechanism. The luminal diameter at this point varies somewhat, depending on the distention of the esophagus by the passage of food, but has been measured at 1.6 to 1.9 cm. These normal constrictions tend to hold up swallowed foreign objects, and the overlying mucosa is subject to injury by swallowed corrosive liquids due to their slow passage through these areas.
Figure 25-3 shows the average distance in centimeters measured during endoscopic examination between the incisor teeth and the cricopharyngeus, aortic arch, and cardia of the stomach. Manometrically, the length of the esophagus between the lower border of the cricopharyngeus and upper border of the lower sphincter varies according to the height of the individual.
The pharyngeal musculature consists of three broad, flat, overlapping fan-shaped constrictors (Fig. 25-4). The opening of the esophagus is collared by the cricopharyngeal muscle, which arises from both sides of the cricoid cartilage of the larynx and forms a continuous transverse muscle band without an interruption by a median raphe. The fibers of this muscle blend inseparably with those of the inferior pharyngeal constrictor above and the inner circular muscle fibers of the esophagus below. Some investigators believe that the cricopharyngeus is part of the inferior constrictor; that is, that the inferior constrictor has two parts, an upper or retrothyroid portion having diagonal fibers, and a lower or retrocricoid portion having transverse fibers. Keith in 1910 showed that these two parts of the same muscle serve totally different functions. The retrocricoid portion serves as the upper sphincter of the esophagus and relaxes when the retrothyroid portion contracts, to force the swallowed bolus from the pharynx into the esophagus.
Figure 25-4.
External muscles of the pharynx. A. Posterolateral view. B. Posterior view. Dotted line represents usual site of myotomy. (Reproduced with permission from Rothberg M, DeMeester TR: Surgical anatomy of the esophagus, in Shields TW (ed): General Thoracic Surgery, 3rd ed. Philadelphia: Lea & Febiger, 1989, p 78.)
The cervical portion of the esophagus is approximately 5 cm long and descends between the trachea and the vertebral column, from the level of the sixth cervical vertebra to the level of the interspace between the first and second thoracic vertebrae posteriorly, or the level of the suprasternal notch anteriorly. The recurrent laryngeal nerves lie in the right and left grooves between the trachea and the esophagus. The left recurrent nerve lies somewhat closer to the esophagus than the right, owing to the slight deviation of the esophagus to the left, and the more lateral course of the right recurrent nerve around the right subclavian artery. Laterally, on the left and right sides of the cervical esophagus are the carotid sheaths and the lobes of the thyroid gland.
The thoracic portion of the esophagus is approximately 20 cm long. It starts at the thoracic inlet. In the upper portion of the thorax, it is in intimate relationship with the posterior wall of the trachea and the prevertebral fascia. Just above the tracheal bifurcation, the esophagus passes to the right of the aorta. This anatomic positioning can cause a notch indentation in its left lateral wall on a barium swallow radiogram. Immediately below this notch, the esophagus crosses both the bifurcation of the trachea and the left main stem bronchus, owing to the slight deviation of the terminal portion of the trachea to the right by the aorta (Fig. 25-5). From there down, the esophagus passes over the posterior surface of the subcarinal lymph nodes (LNs), and then descends over the pericardium of the left atrium to reach the diaphragmatic hiatus (Fig. 25-6). From the bifurcation of the trachea downward, both the vagal nerves and the esophageal nerve plexus lie on the muscular wall of the esophagus.
Figure 25-5.
A. Cross-section of the thorax at the level of the tracheal bifurcation. B. Computed tomographic scan at same level viewed from above: (a) ascending aorta, (b) descending aorta, (c) tracheal carina, (d) esophagus, (e) pulmonary artery. (Reproduced with permission from Rothberg M, DeMeester TR: Surgical anatomy of the esophagus, in Shields TW (ed): General Thoracic Surgery, 3rd ed. Philadelphia: Lea & Febiger, 1989, p 81.)
Figure 25-6.
A. Cross-section of the thorax at the midleft atrial level. B. Computed tomographic scan at same level viewed from above: (a) aorta, (b) esophagus, (c) left atrium, (d) right atrium, (e) left ventricle, (f) right ventricle, (g) pulmonary vein. (Reproduced with permission from Rothberg M, DeMeester TR: Surgical anatomy of the esophagus, in Shields TW (ed): General Thoracic Surgery, 3rd ed. Philadelphia: Lea & Febiger, 1989, p 82.)
Dorsally, the thoracic esophagus follows the curvature of the spine and remains in close contact with the vertebral bodies. From the eighth thoracic vertebra downward, the esophagus moves vertically away from the spine to pass through the hiatus of the diaphragm. The thoracic duct passes through the hiatus of the diaphragm on the anterior surface of the vertebral column behind the aorta and under the right crus. In the thorax, the thoracic duct lies dorsal to the esophagus between the azygos vein on the right and the descending thoracic aorta on the left.
The abdominal portion of the esophagus is approximately 2 cm long and includes a portion of the lower esophageal sphincter (LES). It starts as the esophagus passes through the diaphragmatic hiatus and is surrounded by the phrenoesophageal membrane, a fibroelastic ligament arising from the subdiaphragmatic fascia as a continuation of the transversalis fascia lining the abdomen (Fig. 25-7). The upper leaf of the membrane attaches itself in a circumferential fashion around the esophagus, about 1 to 2 cm above the level of the hiatus. These fibers blend in with the elastic-containing adventitia of the abdominal esophagus and the cardia of the stomach. This portion of the esophagus is subjected to the positive-pressure environment of the abdomen.
Figure 25-7.
Attachments and structure of the phrenoesophageal membrane. Transversalis fascia lies just above the parietal peritoneum. (Reproduced with permission from Rothberg M, DeMeester TR: Surgical anatomy of the esophagus, in Shields TW (ed): General Thoracic Surgery, 3rd ed. Philadelphia: Lea & Febiger, 1989, p 83.)
The musculature of the esophagus can be divided into an outer longitudinal and an inner circular layer. The upper 2 to 6 cm of the esophagus contains only striated muscle fibers. From then on, smooth muscle fibers gradually become more abundant. Most clinically significant esophageal motility disorders involve only the smooth muscle in the lower two- thirds of the esophagus. When a surgical esophageal myotomy is indicated, the incision needs to extend only this distance.
The longitudinal muscle fibers originate from a cricoesophageal tendon arising from the dorsal upper edge of the anteriorly located cricoid cartilage. The two bundles of muscle diverge and meet in the midline on the posterior wall of the esophagus about 3 cm below the cricoid (see Fig. 25-4). From this point on, the entire circumference of the esophagus is covered by a layer of longitudinal muscle fibers. This configuration of the longitudinal muscle fibers around the most proximal part of the esophagus leaves a V-shaped area in the posterior wall covered only with circular muscle fibers. Contraction of the longitudinal muscle fibers shortens the esophagus. The circular muscle layer of the esophagus is thicker than the outer longitudinal layer. In situ, the geometry of the circular muscle is helical and makes the peristalsis of the esophagus assume a worm-like drive, as opposed to segmental and sequential squeezing. As a consequence, severe motor abnormalities of the esophagus assume a corkscrew-like pattern on the barium swallow radiogram.
The cervical portion of the esophagus receives its main blood supply from the inferior thyroid artery. The thoracic portion receives its blood supply from the bronchial arteries, with 75% of individuals having one right-sided and two left-sided branches. Two esophageal branches arise directly from the aorta. The abdominal portion of the esophagus receives its blood supply from the ascending branch of the left gastric artery and from inferior phrenic arteries (Fig. 25-8). On entering the wall of the esophagus, the arteries assume a T-shaped division to form a longitudinal plexus, giving rise to an intramural vascular network in the muscular and submucosal layers. As a consequence, the esophagus can be mobilized from the stomach to the level of the aortic arch without fear of devascularization and ischemic necrosis. Caution should be exercised as to the extent of esophageal mobilization in patients who have had a previous thyroidectomy with ligation of the inferior thyroid arteries proximal to the origin of the esophageal branches.
Blood from the capillaries of the esophagus flows into a submucosal venous plexus, and then into a periesophageal venous plexus from which the esophageal veins originate. In the cervical region, the esophageal veins empty into the inferior thyroid vein; in the thoracic region, they empty into the bronchial, azygos, or hemiazygos veins; and in the abdominal region, they empty into the coronary vein (Fig. 25-9). The submucosal venous networks of the esophagus and stomach are in continuity with each other, and, in patients with portal venous obstruction, this communication functions as a collateral pathway for portal blood to enter the superior vena cava via the azygos vein.
The parasympathetic innervation of the pharynx and esophagus is provided mainly by the vagus nerves. The constrictor muscles of the pharynx receive branches from the pharyngeal plexus, which is on the posterior lateral surface of the middle constrictor muscle, and is formed by pharyngeal branches of the vagus nerves with a small contribution from cranial nerves IX and XI (Fig. 25-10). The cricopharyngeal sphincter and the cervical portion of the esophagus receive branches from both recurrent laryngeal nerves, which originate from the vagus nerves—the right recurrent nerve at the lower margin of the subclavian artery and the left at the lower margin of the aortic arch. They are slung dorsally around these vessels and ascend in the groove between the esophagus and trachea, giving branches to each. Damage to these nerves interferes not only with the function of the vocal cords, but also with the function of the cricopharyngeal sphincter and the motility of the cervical esophagus, predisposing the individual to pulmonary aspiration on swallowing.
Afferent visceral sensory pain fibers from the esophagus end without synapse in the first four segments of the thoracic spinal cord, using a combination of sympathetic and vagal pathways. These pathways are also occupied by afferent visceral sensory fibers from the heart; hence, both organs have similar symptomatology.
The lymphatics located in the submucosa of the esophagus are so dense and interconnected that they constitute a single plexus (Fig. 25-11). There are more lymph vessels than blood capillaries in the submucosa. Lymph flow in the submucosal plexus runs in a longitudinal direction, and, on injection of a contrast medium, the longitudinal spread is seen to be about six times that of the transverse spread. In the upper two-thirds of the esophagus, the lymphatic flow is mostly cephalad, and, in the lower third, caudad. In the thoracic portion of the esophagus, the submucosal lymph plexus extends over a long distance in a longitudinal direction before penetrating the muscle layer to enter lymph vessels in the adventitia. As a consequence of this nonsegmental lymph drainage, a primary tumor can extend for a considerable length superiorly or inferiorly in the submucosal plexus. Consequently, free tumor cells can follow the submucosal lymphatic plexus in either direction for a long distance before they pass through the muscularis and on into the regional LNs. The cervical esophagus has more direct segmental lymph drainage into the regional nodes, and, as a result, lesions in this portion of the esophagus have less submucosal extension and a more regionalized lymphatic spread.
The efferent lymphatics from the cervical esophagus drain into the paratracheal and deep cervical LNs, and those from the upper thoracic esophagus empty mainly into the paratracheal LNs. Efferent lymphatics from the lower thoracic esophagus drain into the subcarinal nodes and nodes in the inferior pulmonary ligaments. The superior gastric nodes receive lymph not only from the abdominal portion of the esophagus, but also from the adjacent lower thoracic segment.
PHYSIOLOGY
The act of alimentation requires the passage of food and drink from the mouth into the stomach. One-third of this distance consists of the mouth and hypopharynx, and two-thirds is made up by the esophagus. To comprehend the mechanics of alimentation, it is useful to visualize the gullet as a mechanical model in which the tongue and pharynx function as a piston pump with three valves, and the body of the esophagus and cardia function as a worm-drive pump with a single valve. The three valves in the pharyngeal cylinder are the soft palate, epiglottis, and cricopharyngeus. The valve of the esophageal pump is the LES. Failure of the valves or the pumps leads to abnormalities in swallowing—that is, difficulty in food propulsion from mouth to stomach—or regurgitation of gastric contents into the esophagus or pharynx.
Food is taken into the mouth in a variety of bite sizes, where it is broken up, mixed with saliva, and lubricated. Once initiated, swallowing is entirely a reflex act. When food is ready for swallowing, the tongue, acting like a piston, moves the bolus into the posterior oropharynx and forces it into the hypopharynx (Fig. 25-12). Concomitantly with the posterior movement of the tongue, the soft palate is elevated, thereby closing the passage between the oropharynx and nasopharynx. This partitioning prevents pressure generated in the oropharynx from being dissipated through the nose. When the soft palate is paralyzed, for example, after a cerebrovascular accident, food is commonly regurgitated into the nasopharynx. During swallowing, the hyoid bone moves upward and anteriorly, elevating the larynx and opening the retrolaryngeal space, bringing the epiglottis under the tongue (see Fig. 25-12). The backward tilt of the epiglottis covers the opening of the larynx to prevent aspiration. The entire pharyngeal part of swallowing occurs within 1.5 seconds.
Figure 25-12.
Sequence of events during the oropharyngeal phase of swallowing. (Reproduced with permission from DeMeester TR, Stein HJ, Fuchs KH: Physiologic diagnostic studies, in Zuidema GD, Orringer MB (eds): Shackelford’s Surgery of the Alimentary Tract, 3rd ed., Vol. I. Philadelphia: W.B. Saunders, 1991, p 95. Copyright Elsevier.)
During swallowing, the pressure in the hypopharynx rises abruptly, to at least 60 mmHg, due to the backward movement of the tongue and contraction of the posterior pharyngeal constrictors. A sizable pressure difference develops between the hypopharyngeal pressure and the less-than-atmospheric midesophageal or intrathoracic pressure (Fig. 25-13). This pressure gradient speeds the movement of food from the hypopharynx into the esophagus when the cricopharyngeus or upper esophageal sphincter relaxes. The bolus is both propelled by peristaltic contraction of the posterior pharyngeal constrictors and sucked into the thoracic esophagus. Critical to receiving the bolus is the compliance of the cervical esophagus; when compliance is lost due to muscle pathology, dysphagia can result. The upper esophageal sphincter closes within 0.5 second of the initiation of the swallow, with the immediate closing pressure reaching approximately twice the resting level of 30 mmHg. The postrelaxation contraction continues down the esophagus as a peristaltic wave (Fig. 25-14). The high closing pressure and the initiation of the peristaltic wave prevents reflux of the bolus from the esophagus back into the pharynx. After the peristaltic wave has passed farther down the esophagus, the pressure in the upper esophageal sphincter returns to its resting level.
Figure 25-13.
Resting pressure profile of the foregut showing the pressure differential between the atmospheric pharyngeal pressure (P) and the less-than-atmospheric midesophageal pressure (E) and greater-than-atmospheric intragastric pressure (G), with the interposed high pressure zones of the cricopharyngeus (C) and distal esophageal sphincter (DES). The necessity for relaxation of the cricopharyngeus and DES pressure to move a bolus into the stomach is apparent. Esophageal work occurs when a bolus is pushed from the midesophageal area (E), with a pressure less than atmospheric, into the stomach, which has a pressure greater than atmospheric (G). (Reproduced with permission from Waters PF, DeMeester TR: Foregut motor disorders and their surgical management. Med Clin North Am 65:1237, 1981. Copyright Elsevier.)
Swallowing can be started at will, or it can be reflexively elicited by the stimulation of areas in the mouth and pharynx, among them the anterior and posterior tonsillar pillars or the posterior lateral walls of the hypopharynx. The afferent sensory nerves of the pharynx are the glossopharyngeal nerves and the superior laryngeal branches of the vagus nerves. Once aroused by stimuli entering via these nerves, the swallowing center in the medulla coordinates the complete act of swallowing by discharging impulses through cranial nerves V, VII, X, XI, and XII, as well as the motor neurons of C1 to C3. Discharges through these nerves occur in a rather specific pattern and last for approximately 0.5 second. Little is known about the organization of the swallowing center, except that it can trigger swallowing after a variety of different inputs, but the response is always a rigidly ordered pattern of outflow. Following a cerebrovascular accident, this coordinated outflow may be altered, causing mild to severe abnormalities of swallowing. In more severe injury, swallowing can be grossly disrupted, leading to repetitive aspiration.
The striated muscles of the cricopharyngeus and the upper one-third of the esophagus are activated by efferent motor fibers distributed through the vagus nerve and its recurrent laryngeal branches. The integrity of innervation is required for the cricopharyngeus to relax in coordination with the pharyngeal contraction, and resume its resting tone once a bolus has entered the upper esophagus. Operative damage to the innervation can interfere with laryngeal, cricopharyngeal, and upper esophageal function, and predispose the patient to aspiration.
The pharyngeal activity in swallowing initiates the esophageal phase. The body of the esophagus functions as a worm-drive propulsive pump due to the helical arrangement of its circular muscles, and is responsible for transferring a bolus of food into the stomach. The esophageal phases of swallowing represents esophageal work done during alimentation, in that food is moved into the stomach from a negative-pressure environment of –6 mm Hg intrathoracic pressure, to a positive-pressure environment of 6 mm Hg intra-abdominal pressure, or over a gradient of 12 mm Hg (see Fig. 25-13). Effective and coordinated smooth muscle function in the lower one- third of the esophagus is therefore important in pumping the food across this gradient.
The peristaltic wave generates an occlusive pressure varying from 30 to 120 mmHg (see Fig. 25-14). The wave rises to a peak in 1 second, lasts at the peak for about 0.5 second, and then subsides in about 1.5 seconds. The whole course of the rise and fall of occlusive pressure may occupy one point in the esophagus for 3 to 5 seconds. The peak of a primary peristaltic contraction initiated by a swallow (primary peristalsis) moves down the esophagus at 2 to 4 cm/s and reaches the distal esophagus about 9 seconds after swallowing starts. Consecutive swallows produce similar primary peristaltic waves, but when the act of swallowing is rapidly repeated, the esophagus remains relaxed and the peristaltic wave occurs only after the last movement of the pharynx. Progress of the wave in the esophagus is caused by sequential activation of its muscles, initiated by efferent vagal nerve fibers arising in the swallowing center.
Continuity of the esophageal muscle is not necessary for sequential activation if the nerves are intact. If the muscles, but not the nerves, are cut across, the pressure wave begins distally below the cut as it dies out at the proximal end above the cut. This allows a sleeve resection of the esophagus to be done without destroying its normal function. Afferent impulses from receptors within the esophageal wall are not essential for progress of the coordinated wave. Afferent nerves, however, do go to the swallowing center from the esophagus, because, if the esophagus is distended at any point, a contractual wave begins with a forceful closure of the upper esophageal sphincter and sweeps down the esophagus. This secondary contraction occurs without any movements of the mouth or pharynx. Secondary peristalsis can occur as an independent local reflex to clear the esophagus of ingested material left behind after the passage of the primary wave. Current studies suggest that secondary peristalsis is not as common as once thought.
Despite the powerful occlusive pressure, the propulsive force of the esophagus is relatively feeble. If a subject attempts to swallow a bolus attached by a string to a counterweight, the maximum weight that can be overcome is 5 to 10 g. Orderly contractions of the muscular wall and anchoring of the esophagus at its inferior end are necessary for efficient aboral propulsion to occur. Loss of the inferior anchor, as occurs with a large hiatal hernia, can lead to inefficient propulsion.
The LES provides a pressure barrier between the esophagus and stomach and acts as the valve on the worm-drive pump of the esophageal body. Although an anatomically distinct LES has been difficult to identify, microdissection studies show that, in humans, the sphincter-like function is related to the architecture of the muscle fibers at the junction of the esophageal tube with the gastric pouch (Fig. 25-15). The sphincter actively remains closed to prevent reflux of gastric contents into the esophagus and opens by a relaxation that coincides with a pharyngeal swallow (see Fig. 25-14). The LES pressure returns to its resting level after the peristaltic wave has passed through the esophagus. Consequently, reflux of gastric juice that may occur through the open valve during a swallow is cleared back into the stomach.
Figure 25-15.
Wall thickness and orientation of fibers on microdissection of the cardia. At the junction of the esophageal tube and gastric pouch, there is an oblique muscular ring composed of an increased muscle mass inside the inner muscular layer. On the lesser curve side of the cardia, the muscle fibers of the inner layer are oriented transversely and form semicircular muscle clasps. On the greater curve side of the cardia, these muscle fibers form oblique loops that encircle the distal end of the cardia and gastric fundus. Both the semicircular muscle clasps and the oblique fibers of the fundus contract in a circular manner to close the cardia. (Reproduced with permission from DeMeester TR, Skinner DB: Evaluation of esophageal function and disease, in Glenn WWL (ed): Thoracic and Cardiovascular Surgery, 4th ed. Norwalk, CT: Appleton-Century-Crofts, 1983, p 461.)
If the pharyngeal swallow does not initiate a peristaltic contraction, then the coincident relaxation of the LES is unguarded and reflux of gastric juice can occur. This may be an explanation for the observation of spontaneous lower esophageal relaxation, thought by some to be a causative factor in gastroesophageal reflux disease (GERD). The power of the worm-drive pump of the esophageal body is insufficient to force open a valve that does not relax. In dogs, a bilateral cervical parasympathetic blockade abolishes the relaxation of the LES that occurs with pharyngeal swallowing or distention of the esophagus. Consequently, vagal function appears to be important in coordinating the relaxation of the LES with esophageal contraction.
The antireflux mechanism in human beings is composed of three components: a mechanically effective LES, efficient esophageal clearance, and an adequately functioning gastric reservoir. A defect of any one of these three components can lead to increased esophageal exposure to gastric juice and the development of mucosal injury.
On 24-hour esophageal pH monitoring, healthy individuals have occasional episodes of gastroesophageal reflux. This physiologic reflux is more common when awake and in the upright position than during sleep in the supine position. When reflux of gastric juice occurs, normal subjects rapidly clear the acid gastric juice from the esophagus regardless of their position.
There are several explanations for the observation that physiologic reflux in normal subjects is more common when they are awake and in the upright position than during sleep in the supine position. First, reflux episodes occur in healthy volunteers primarily during transient losses of the gastroesophageal barrier, which may be due to a relaxation of the LES or intragastric pressure overcoming sphincter pressure. Gastric juice can also reflux when a swallow-induced relaxation of the LES is not protected by an oncoming peristaltic wave. The average frequency of these “unguarded moments” or of transient losses of the gastroesophageal barrier is far less while asleep and in the supine position than while awake and in the upright position. Consequently, there are fewer opportunities for reflux to occur in the supine position. Second, in the upright position, there is a 12-mmHg pressure gradient between the resting, positive intra-abdominal pressure measured in the stomach and the most negative intrathoracic pressure measured in the esophagus at midthoracic level. This gradient favors the flow of gastric juice up into the thoracic esophagus when upright. The gradient diminishes in the supine position. Third, the LES pressure in normal subjects is significantly higher in the supine position than in the upright position. This is due to the apposition of the hydrostatic pressure of the abdomen to the abdominal portion of the sphincter when supine. In the upright position, the abdominal pressure surrounding the sphincter is negative compared with atmospheric pressure, and, as expected, the abdominal pressure gradually increases the more caudally it is measured. This pressure gradient tends to move the gastric contents toward the cardia and encourages the occurrence of reflux into the esophagus when the individual is upright. In contrast, in the supine position, the gastroesophageal pressure gradient diminishes, and the abdominal hydrostatic pressure under the diaphragm increases, causing an increase in sphincter pressure and a more competent cardia.
The LES has intrinsic myogenic tone, which is modulated by neural and hormonal mechanisms. Alpha-adrenergic neurotransmitters or beta blockers stimulate the LES, and alpha blockers and beta stimulants decrease its pressure. It is not clear to what extent cholinergic nerve activity controls LES pressure. The vagus nerve carries both excitatory and inhibitory fibers to the esophagus and sphincter. The hormones gastrin and motilin have been shown to increase LES pressure; and cholecystokinin, estrogen, glucagon, progesterone, somatostatin, and secretin decrease LES pressure. The peptides bombesin, l-enkephalin, and substance P increase LES pressure; and calcitonin gene-related peptide, gastric inhibitory peptide, neuropeptide Y, and vasoactive intestinal polypeptide decrease LES pressure. Some pharmacologic agents such as antacids, cholinergics, agonists, domperidone, metoclopramide, and prostaglandin F2 are known to increase LES pressure; and anticholinergics, barbiturates, calcium channel blockers, caffeine, diazepam, dopamine, meperidine, prostaglandin E1 and E2, and theophylline decrease LES pressure. Peppermint, chocolate, coffee, ethanol, and fat are all associated with decreased LES pressure and may be responsible for esophageal symptoms after a sumptuous meal.
ASSESSMENT OF ESOPHAGEAL FUNCTION
A thorough understanding of the patient’s underlying anatomic and functional deficits before making therapeutic decisions is fundamental to the successful treatment of esophageal disease. The diagnostic tests, as presently used, may be divided into four broad groups: (a) tests to detect structural abnormalities of the esophagus; (b) tests to detect functional abnormalities of the esophagus; (c) tests to detect increased esophageal exposure to gastric juice; and (d) tests of duodenogastric function as they relate to esophageal disease.
The first diagnostic test in patients with suspected esophageal disease is usually upper gastrointestinal endoscopy. This allows assessment and biopsy of the mucosa of the stomach and the esophagus, as well as the diagnosis and assessment of obstructing lesions in the upper gastrointestinal tract. In any patient complaining of dysphagia, esophagoscopy is indicated, even in the face of a normal radiographic study.
For the initial endoscopic assessment, the flexible fiber-optic esophagoscope is the instrument of choice because of its technical ease, patient acceptance, and the ability to simultaneously assess the stomach and duodenum. Rigid endoscopy is now only rarely required, mainly for the disimpaction of difficult foreign bodies impacted in the esophagus.
When GERD is the suspected diagnosis, particular attention should be paid to detecting the presence of esophagitis and Barrett’s columnar-lined esophagus (CLE). When endoscopic esophagitis is seen, severity and the length of esophagitis involved are recorded. Many different grading systems have been proposed. In a commonly used system, Grade I esophagitis is defined as small, circular, nonconfluent erosions (Fig. 25-16). Grade II esophagitis is defined by the presence of linear erosions lined with granulation tissue that bleeds easily when touched. Grade III esophagitis represents a more advanced stage, in which the linear erosions coalesce into circumferential loss of the epithelium and the mucosa may take a “cobblestone” appearance. Grade IV esophagitis is the presence of a stricture. Its severity can be assessed by the ease of passing a 36F endoscope. When a stricture is observed, the severity of the esophagitis above it should be recorded. The absence of esophagitis above a stricture suggests a chemical-induced injury or a neoplasm as a cause. The latter should always be considered and is ruled out only by evaluation of a tissue biopsy of adequate size. It should be remembered that gastroesophageal reflux is not always associated with visible mucosal abnormalities, and patients can experience significant reflux symptoms, despite an apparently normal endoscopy examination.
Barrett’s esophagus (BE) is a condition in which the tubular esophagus is lined with columnar epithelium, as opposed to the normal squamous epithelium (Fig. 25-16). Histologically, it appears as intestinal metaplasia (IM). It is suspected at endoscopy when there is difficulty in visualizing the squamocolumnar junction at its normal location, and by the appearance of a redder, more luxuriant mucosa than is normally seen in the lower esophagus. Its presence is confirmed by biopsy. Multiple biopsy specimens should be taken in a cephalad direction to determine the level at which the junction of Barrett’s epithelium with normal squamous mucosa occurs. BE is susceptible to ulceration, bleeding, stricture formation, and, most important, malignant degeneration. The earliest sign of the latter is high grade dysplasia or intramucosal adenocarcinoma (Fig. 25-16). These dysplastic changes have a patchy distribution, so a minimum of four biopsy samples spaced 2 cm apart should be taken from the Barrett’s-lined portion of the esophagus. Changes seen in one biopsy are significant. Nishimaki has determined that the tumors occur in an area of specialized columnar epithelium near the squamocolumnar junction in 85% of patients, and within 2 cm of the squamocolumnar junction in virtually all patients. Particular attention should be focused on this area in patients suspected of harboring a carcinoma.
Abnormalities of the gastroesophageal flap valve can be visualized by retroflexion of the endoscope. Hill has graded the appearance of the gastroesophageal valve from I to IV according to the degree of unfolding or deterioration of the normal valve architecture (Fig. 25-17). The appearance of the valve correlates with the presence of increased esophageal acid exposure, occurring predominantly in patients with Grade III and IV valves.
Figure 25-17.
A. Grade I flap valve appearance. Note the ridge of tissue that is closely approximated to the shaft of the retroflexed endoscope. It extends 3–4 cm along the lesser curve. B. Grade II flap valve appearance. The ridge is slightly less well defined than in grade I and it opens rarely with respiration and closes promptly. C. Grade III flap valve appearance. The ridge is barely present, and there is often failure to close around the endoscope. It is nearly always accompanied by a hiatal hernia. D. Grade IV flap valve appearance. There is no muscular ridge at all. The gastroesophageal valve stays open all the time, and squamous epithelium can often be seen from the retroflexed position. A hiatal hernia is always present. (Reproduced with permission from Hill LD, Kozarek RA, et al.: The gastroesophageal flap valve. In vitro and in vivo observations. Gastrointest Endosc. 44:541, 1996. Copyright Elsevier.)
A hiatal hernia is endoscopically confirmed by finding a pouch lined with gastric rugal folds lying 2 cm or more above the margins of the diaphragmatic crura, identified by having the patient sniff. A hernia is best demonstrated with the stomach fully insufflated and the gastroesophageal junction observed with a retroflexed endoscope. A prominent sliding hiatal hernia frequently is associated with increased esophageal exposure to gastric juice. When a paraesophageal hernia (PEH) is observed, particular attention is taken to exclude a gastric (Cameron’s) ulcer or gastritis within the pouch. The intragastric retroflex or J maneuver is important in evaluating the full circumference of the mucosal lining of the herniated stomach.
When an esophageal diverticulum is seen, it should be carefully explored with the flexible endoscope to exclude ulceration or neoplasia. When a submucosal mass is identified, biopsy specimens are usually not performed. At the time of surgical resection, a submucosal leiomyoma or reduplication cyst can generally be dissected away from the intact mucosa, but if a biopsy sample is taken, the mucosa may become fixed to the underlying abnormality. This complicates the surgical dissection by increasing the risk of mucosal perforation. Endoscopic ultrasound provides a better method for evaluating these lesions.
Barium swallow evaluation is undertaken selectively to assess anatomy and motility. The anatomy of large hiatal hernias are more clearly demonstated by contrast radiology than endoscopy, and the presence of coordinated esophageal peristalsis can be determined by observing several individual swallows of barium traversing the entire length of the organ, with the patient in the horizontal position. Hiatal hernias are best demonstrated with the patient prone because the increased intra-abdominal pressure produced in this position promotes displacement of the esophagogastric junction above the diaphragm. To detect lower esophageal narrowing, such as rings and strictures, fully distended views of the esophagogastric region are crucial. The density of the barium used to study the esophagus can potentially affect the accuracy of the examination. Esophageal disorders shown clearly by a full-column technique include circumferential carcinomas, peptic strictures, large esophageal ulcers, and hiatal hernias. A small hiatal hernia is usually not associated with significant symptoms or illness, and its presence is an irrelevant finding unless the hiatal hernia is large (Fig. 25-18), or the hernia is of the paraesophageal variety. Lesions extrinsic but adjacent to the esophagus can be reliably detected by the full-column technique if they contact the distended esophageal wall. Conversely, a number of important disorders may go undetected if this is the sole technique used to examine the esophagus. These include small esophageal neoplasms, mild esophagitis, and esophageal varices. Thus, the full-column technique should be supplemented with mucosal relief or double-contrast films to enhance detection of these smaller or more subtle lesions.
Motion-recording techniques greatly aid in evaluating functional disorders of the pharyngoesophageal and esophageal phases of swallowing. The technique and indications for cine- and videoradiography will be discussed in the section entitled Video- and Cineradiography, as they are more useful to evaluate function and seldom used to detect structural abnormalities.
The radiographic assessment of the esophagus is not complete unless the entire stomach and duodenum have been examined. A gastric or duodenal ulcer, partially obstructing gastric neoplasm, or scarred duodenum and pylorus may contribute significantly to symptoms otherwise attributable to an esophageal abnormality.
When a patient’s complaints include dysphagia and no obstructing lesion is seen on the barium swallow, it is useful to have the patient swallow a barium-impregnated marshmallow, a barium-soaked piece of bread, or a hamburger mixed with barium. This test may bring out a functional disturbance in esophageal transport that can be missed when liquid barium is used.
In many patients with symptoms of an esophageal disorder, standard radiographic and endoscopic evaluation fails to demonstrate a structural abnormality. In these situations, esophageal function tests are necessary to identify a functional disorder.
Esophageal manometry is a widely used technique to examine the motor function of the esophagus and its sphincters. Manometry is indicated whenever a motor abnormality of the esophagus is suspected on the basis of complaints of dysphagia, odynophagia, or noncardiac chest pain, and the barium swallow or endoscopy does not show a clear structural abnormality. Esophageal manometry is particularly necessary to confirm the diagnosis of specific primary esophageal motility disorders (i.e., achalasia, diffuse esophageal spasm [DES], nutcracker esophagus, and hypertensive LES). It also identifies nonspecific esophageal motility abnormalities and motility disorders secondary to systemic disease such as scleroderma, dermatomyositis, polymyositis, or mixed connective tissue disease. In patients with symptomatic GERD, manometry of the esophageal body can identify a mechanically defective LES, and evaluate the adequacy of esophageal peristalsis and contraction amplitude. Manometry has become an essential tool in the preoperative evaluation of patients before antireflux surgery, guiding selection of the appropriate procedure based upon the patient’s underlying esophageal function, and excluding patients with achalasia who can be misdiagnosed with gastroesophageal reflux when clinical and endoscopic parameters alone are used for diagnosis.
Esophageal manometry is performed using electronic, pressure-sensitive transducers located within the catheter, or water-perfused catheters with lateral side holes attached to transducers outside the body. The traditional catheter consists of a train of five pressure transducers or five or more water-perfused tubes bound together. The transducers or lateral openings are placed at 5-cm intervals from the tip and oriented radially at 72° from each other around the circumference of the catheter. A special catheter assembly consisting of four lateral openings at the same level, oriented at 90° to each other, is of special use in measuring the three-dimensional vector volume of the LES. Other specially designed catheters can be used to assess the upper sphincter.
As the pressure-sensitive station is brought across the gastroesophageal junction (GEJ), a rise in pressure above the gastric baseline signals the beginning of the LES. The respiratory inversion point is identified when the positive excursions that occur in the abdominal cavity with breathing change to negative deflections in the thorax. The respiratory inversion point serves as a reference point at which the amplitude of LES pressure and the length of the sphincter exposed to abdominal pressure are measured. As the pressure-sensitive station is withdrawn into the body of the esophagus, the upper border of the LES is identified by the drop in pressure to the esophageal baseline. From these measurements, the pressure, abdominal length, and overall length of the sphincter are determined (Fig. 25-19). To account for the asymmetry of the sphincter (Fig. 25-20), the pressure profile is repeated with each of the five radially oriented transducers, and the average values for sphincter pressure above gastric baseline, overall sphincter length, and abdominal length of the sphincter are calculated.
Figure 25-20.
Radial configuration of the lower esophageal sphincter. A = anterior; L = left; LA = left anterior; LP = left posterior; P = posterior; R = right; RA = right anterior; RP = right posterior. (Reproduced with permission from Winans CS: Manometric asymmetry of the lower esophageal high pressure zone. Dig Dis Sci 22:348, 1977. With kind permission from Springer Science+Business Media.)
Table 25-1 shows the values for these parameters in 50 normal volunteers without subjective or objective evidence of a foregut disorder. The level at which a deficiency in the mechanics of the LES occurs was defined by comparing the frequency distribution of these values in the 50 healthy volunteers with a population of similarly studied patients with symptoms of GERD. The presence of increased esophageal exposure to gastric juice was documented by 24-hour esophageal pH monitoring. Based on these studies, a mechanically defective sphincter is identified by having one or more of the following characteristics: an average LES pressure of <6 mmHg, an average length exposed to the positive-pressure environment in the abdomen of 1 cm or less, and/or an average overall sphincter length of 2 cm or less. Compared with the normal volunteers, these values are below the 2.5 percentile for sphincter pressure and overall length and for abdominal length.
MEDIAN | PERCENTILE | ||
---|---|---|---|
2.5 | 97.5 | ||
Pressure (mmHg) | 13 | 5.8 | 27.7 |
Overall length (cm) | 3.6 | 2.1 | 5.6 |
Abdominal length (cm) | 2 | 0.9 | 4.7 |
Mean | Mean – 2 SD | Mean + 2 SD | |
Pressure (mmHg) | 13.8 ± 4.6 | 4.6 | 23.0 |
Overall length (cm) | 3.7 ± 0.8 | 2.1 | 5.3 |
Abdominal length (cm) | 2.2 ± 0.8 | 0.6 | 3.8 |
To assess the relaxation and postrelaxation contraction of the LES, a pressure transducer is positioned within the high-pressure zone, with the distal transducer located in the stomach and the proximal transducer within the esophageal body. Ten wet swallows (5 mL water each) are performed. The normal pressure of the LES should drop to the level of gastric pressure during each wet swallow.
The function of the esophageal body is assessed with the five pressure transducers located in the esophagus. The standard procedure is to locate the most proximal pressure transducer 1 cm below the well-defined cricopharyngeal sphincter, allowing a pressure response throughout the whole esophagus to be obtained on one swallow. Ten wet swallows are recorded. Amplitude, duration, and morphology of contractions following each swallow are calculated at all recorded levels of the esophageal body. The delay between the onset and peak of esophageal contractions at the various levels of the esophagus is used to calculate the speed of wave propagation. The relationship of the esophageal contractions following a swallow is classified as peristaltic or simultaneous. The data are used to identify motor disorders of the esophagus.
The position, length, and pressure of the cricopharyngeal sphincter are assessed with a stationary pull-through technique similar to that used for the LES. The manometric catheter is withdrawn in 0.5-cm intervals from the upper esophagus through the upper esophageal sphincter region into the pharynx. The relaxation of the upper esophageal sphincter is studied by straddling the eight pressure transducers across the sphincter so that some are in the pharynx and some are in the upper esophagus. High-speed graphic recordings (50 mm/s) are necessary to obtain an assessment of the coordination of cricopharyngeal relaxation with hypopharyngeal contraction. It has been difficult to consistently demonstrate a motility abnormality in patients with pharyngoesophageal disorders.
Esophageal manometry was introduced into clinical practice in the 1970s and, until recently, has changed little. In 1991, Ray Clouse introduced the concept of improving conventional manometry by increasing the number of recording sites and adding a three-dimensional assessment. This “high-resolution manometry” is a variant of the conventional manometry in which multiple, circumferential recording sites are used, in essence creating a “map” of the esophagus and its sphincters. High-resolution catheters contain 36 miniaturized pressure sensors positioned every centimeter along the length of the catheter. The vast amount of data generated by these sensors is then processed and presented in traditional linear plots or as a visually enhanced spatiotemporal video tracing that is readily interpreted (Fig. 25-21).
Simultaneous acquisition of data for the upper esophageal sphincter, esophageal body, LES, and gastric pressure minimizes the movement artifacts and study time associated with conventional esophageal manometry. Powerful, computer-based, and easy-to-use tools give unprecedented data analysis capability. This technology significantly enhances esophageal diagnostics, bringing it into the realm of “image” based studies. High-resolution manometry may allow the identification of focal motor abnormalities previously overlooked. It has enhanced the ability to predict bolus propagation and increased sensitivity in the measurement of pressure gradients.
New technology recently introduced into the clinical realm allows measurement of esophageal function and gastroesophageal reflux in a way that has heretofore not been possible. An intraluminal electrical impedance catheter has recently been developed for the measurement of GI function. Impedance is the ratio of voltage to current, and is a measure of the electrical conductivity of a hollow organ and its contents. Intraluminal electrical impedance is inversely proportional to the electrical conductivity of the luminal contents and the cross-sectional area of the lumen. Air has a very low electrical conductivity and, therefore, high impedance. Saliva and food cause an impedance decrease because of their increased conductivity. Luminal dilatation results in a decrease in impedance, whereas luminal contraction yields an impedance increase. Investigators have established the impedance waveform characteristics that define esophageal bolus transport. This allows for the characterization of both esophageal function, via quantification of bolus transport, and gastroesophageal reflux (Fig. 25-22). The probe measures impedance between adjacent electrodes, with measuring segments located at 2, 4, 6, 8, 14, and 16 cm from the distal tip. An extremely low electric current of 0.00025 μW is transmitted across the electrodes at a frequency of 1 to 2 kHz and is limited to 8 μA. This is below the stimulation threshold for nerves and muscles, and is three orders of magnitude below the threshold of cardiac stimulation. A standard pH electrode is located 5 cm from the distal tip, so that the acidic or nonacidic nature of refluxate can be correlated with the number of reflux events.
Esophageal impedance has been validated as an appropriate method for the evaluation of GI function and is becoming increasingly used for the diagnosis of gastroesophageal reflux. It has been compared to cineradiography showing that impedance waves correspond well with actual bolus transport illustrated by radiography. Bolus entry, transit, and exit can be clearly identified by impedance changes in the corresponding measuring segments. Preliminary studies comparing standard esophageal manometry with impedance measurements in healthy volunteers have been performed, and have validated the ability of esophageal impedance to correlate with peristaltic wave progression and bolus length. Clinical investigators are beginning to examine and validate impedance measurement in the evaluation of esophageal and small intestinal pathophysiology.
It is increasingly recognized that 24-hour pH monitoring as the historical gold standard for diagnosing and quantifying gastroesophageal reflux has significant limitations. With 24-hour ambulatory pH testing, reflux is defined as a drop in the pH below 4, which effectively “blinds” the test to reflux occurring at higher pH values. Furthermore, in patients with persistent symptoms on proton pump inhibitor (PPI) therapy, pH monitoring has limited use as it can only detect abnormal acid reflux (pH <4), the occurrence of which has been altered by the antisecretory medication. Given that PPI antisecretory therapy is highly effective in neutralizing gastric acid, the question of whether persistent symptoms are a result of persistent acid reflux, nonacid reflux, or are not reflux related becomes a key issue in surgical decision making. Until recently, this differentiation could not be made. A reliable method for detecting both acid and nonacid reflux has potential to define these populations of patients and thus improve patient selection for antireflux surgery. The recent introduction of multichannel intraluminal impedance technology allows the measurement of both acid and nonacid reflux, with potential to significantly enhance diagnostic accuracy.
Using this technology, Balaji and colleagues showed that most gastroesophageal reflux remains despite acid suppression. Impedance pH may be particularly useful in evaluating patients with persistent symptoms despite PPI treatment, patients with respiratory symptoms, and postoperative patients who are having symptoms that are elusive to diagnosis.
The esophageal transit of a 10-mL water bolus containing technetium-99m (99mTc) sulfur colloid can be recorded with a gamma camera. Using this technique, delayed bolus transit has been shown in patients with a variety of esophageal motor disorders, including achalasia, scleroderma, DES, and nutcracker esophagus.
High-speed cinematic or video recording of radiographic studies allows re-evaluation by reviewing the studies at various speeds. This technique is more useful than manometry in the evaluation of the pharyngeal phase of swallowing. Observations suggesting oropharyngeal or cricopharyngeal dysfunction include misdirection of barium into the trachea or nasopharynx, prominence of the cricopharyngeal muscle, a Zenker’s diverticulum, a narrow pharyngoesophageal segment, and stasis of the contrast medium in the valleculae or hypopharyngeal recesses (Fig. 25-23). These findings are usually not specific, but rather common manifestations of neuromuscular disorders affecting the pharyngoesophageal area. Studies using liquid barium, barium-impregnated solids, or radiopaque pills aid the evaluation of normal and abnormal motility in the esophageal body. Loss of the normal stripping wave or segmentation of the barium column with the patient in the recumbent position correlates with abnormal motility of the esophageal body. In addition, structural abnormalities such as small diverticula, webs, and minimal extrinsic impressions of the esophagus may be recognized only with motion-recording techniques. The simultaneous computerized capture of videofluoroscopic images and manometric tracings is now available, and is referred to as manofluorography. Manofluorographic studies allow precise correlation of the anatomic events, such as opening of the upper esophageal sphincter, with manometric observations, such as sphincter relaxation. Manofluorography, although not widely available, is presently the best means available to evaluate complex functional abnormalities.
Figure 25-23.
Esophagograms from a patient with cricopharyngeal achalasia. A. Anteroposterior film showing retention of the contrast medium at the level of the vallecula and piriform recesses, with no barium passing into the esophagus. B. Lateral film, taken opposite the C5–C6 vertebrae, showing posterior indentation of the cricopharyngeus, retention in the hypopharynx, and tracheal aspiration. (Reproduced with permission from Lafontaine E: Pharyngeal dysphagia, in DeMeester TR, Matthews H (eds): International Trends in General Thoracic Surgery, Vol. 3. Benign Esophageal Disease. St. Louis: Mosby, 1987, p 345. Copyright Elsevier.)
The most direct method of measuring increased esophageal exposure to gastric juice is by an indwelling pH electrode, or, more recently, via a radiotelemetric pH monitoring capsule that can be clipped to the esophageal mucosa. The latter consists of an antimony pH electrode fitted inside a small, capsule-shaped device accompanied by a battery and electronics that allow 48-hour monitoring and transmission of the pH data via transcutaneous radio telemetry to a waist-mounted data logger. The device can be introduced either transorally or transnasally, and clipped to the esophageal mucosa using endoscopic fastening techniques. It passes spontaneously within 1 to 2 weeks. Prolonged monitoring of esophageal pH is performed by placing the pH probe or telemetry capsule 5 cm above the manometrically measured upper border of the distal sphincter for 24 hours. It measures the actual time the esophageal mucosa is exposed to gastric juice, measures the ability of the esophagus to clear refluxed acid, and correlates esophageal acid exposure with the patient’s symptoms. A 24- to 48-hour period is necessary so that measurements can be made over one or two complete circadian cycles. This allows measuring the effect of physiologic activity, such as eating or sleeping, on the reflux of gastric juice into the esophagus (Fig. 25-24).
Figure 25-24.
Strip chart display of a 24-hour esophageal pH monitoring study in a patient with increased esophageal acid exposure. mp = meal period; sp = supine period. (Reproduced with permission from DeMeester TR, Stein HJ, Fuchs KH: Physiologic diagnostic studies, in Zuidema GD, Orringer MB (eds): Shackelford’s Surgery of the Alimentary Tract, 3rd ed, Vol. I. Philadelphia: W.B. Saunders, 1991, p 119. Copyright Elsevier.)
The 24-hour esophageal pH monitoring should not be considered a test for reflux, but rather a measurement of the esophageal exposure to gastric juice. The measurement is expressed by the time the esophageal pH was below a given threshold during the 24-hour period. This single assessment, although concise, does not reflect how the exposure has occurred; that is, did it occur in a few long episodes or several short episodes? Consequently, two other assessments are necessary: the frequency of the reflux episodes and their duration.
The units used to express esophageal exposure to gastric juice are: (a) cumulative time the esophageal pH is below a chosen threshold, expressed as the percentage of the total, upright, and supine monitored time; (b) frequency of reflux episodes below a chosen threshold, expressed as number of episodes per 24 hours; and (c) duration of the episodes, expressed as the number of episodes >5 minutes per 24 hours, and the time in minutes of the longest episode recorded. Table 25-2 shows the normal values for these components of the 24-hour record at the whole-number pH threshold derived from 50 normal asymptomatic subjects. The upper limits of normal were established at the ninety-fifth percentile. Most centers use pH 4 as the threshold.
The components’ 24-hour pH record are then combined into one expression of the overall esophageal acid exposure below a pH threshold; the pH score is calculated by using the standard deviation (SD) of the mean of each of the six components measured in the 50 normal subjects as a weighting factor. By accepting an abstract zero level two SDs below the mean, the data measured in normal subjects could be treated as though they had a normal distribution. Thus, any measured patient value could be referenced to this zero point, and, in turn, be awarded points based on whether it was below or above the normal mean value for that component, according to this formula:
The upper limits of normal for the composite score for each whole-number pH threshold are shown in Table 25-3.
The detection of increased esophageal exposure to acid gastric juice is more dependable than the detection of increased exposure to alkaline gastric juice. The latter is suggested by an increased alkaline exposure time above pH 7 or 8. Increased exposure in this pH range can be caused by abnormal calibration of the pH recorder; dental infection, which increases salivary pH; esophageal obstruction, which results in static pools of saliva with an increase in pH secondary to bacterial overgrowth; or regurgitation of alkaline gastric juice into the esophagus. Using a properly calibrated probe, in the absence of dental infections or esophageal obstruction, the percentage of time the pH is measured above 7 correlates with the concentration of bile acids continuously aspirated over a 24-hour period.
When done in a test population with an equal distribution of normal healthy subjects and patients with the classic reflux symptoms and a defective sphincter, 24-hour esophageal pH monitoring had a sensitivity and specificity of 96%. (Sensitivity is the ability to detect a disease when known to be present; specificity is the ability to exclude the disease when known to be absent.) This gave a predictive value of a positive and negative test of 96%, and an overall accuracy of 96%. Based on these studies and extensive clinical experience, 24-hour esophageal pH monitoring has emerged as the gold standard for the diagnosis of GERD.
Catheter-based, 24-hour, ambulatory, esophageal pH monitoring does have a significant drawback of the physical presence of a transnasal catheter that must be worn for 24 hours. Although most doctors ask patients to go about their normal activities of daily living, many do not comply due to embarrassment about the catheter or from discomfort. The recent development of a wireless capsule, which can be implanted in the esophagus and record pH data for 48 hours, has significantly changed patient satisfaction with the procedure.
The Bravo pH Capsule (Medtronics, Minneapolis, MN) measures pH levels in the esophagus and transmits continuous esophageal pH readings to a receiver worn on the patient’s belt or waistband (Fig. 25-27). Symptoms that the patient experience are recorded in a diary and/or by pressing buttons on the receiver unit. Generally, 48 hours of pH data are measured with this probe. A recent study has shown that the addition of a second day of pH monitoring increased the sensitivity of pH measurement by 22%. The capsule eventually detaches and passes through the digestive tract in 5 to 7 days.
The definition of radiographic gastroesophageal reflux varies depending on whether reflux is spontaneous or induced by various maneuvers. In only about 40% of patients with classic symptoms of GERD is spontaneous reflux (i.e., reflux of barium from the stomach into the esophagus with the patient in the upright position) observed by the radiologist. In most patients who show spontaneous reflux on radiography, the diagnosis of increased esophageal acid exposure is confirmed by 24-hour esophageal pH monitoring. Therefore, the radiographic demonstration of spontaneous regurgitation of barium into the esophagus in the upright position is a reliable indicator that reflux is present. However, failure to see this does not indicate the absence of disease, and for this reason this test is rarely relied on for clinical diagnosis.
Esophageal disorders are frequently associated with abnormalities of duodenogastric function. Abnormalities of the gastric reservoir or increased gastric acid secretion can be responsible for increased esophageal exposure to gastric juice. Reflux of alkaline duodenal juice, including bile salts, pancreatic enzymes, and bicarbonate, is thought to have a role in the pathogenesis of esophagitis and complicated Barrett’s esophagus. Furthermore, functional disorders of the esophagus are often not confined to the esophagus alone, but are associated with functional disorders of the rest of the foregut (i.e., stomach and duodenum). Tests of duodenogastric function that are helpful to investigate esophageal symptoms include gastric emptying studies, gastric acid analysis, and cholescintigraphy (for the diagnosis of pathologic duodenogastric reflux). The single test of 24-hour gastric pH monitoring can be used to identify gastric hypersecretion and imply the presence of duodenogastric reflux and delayed gastric emptying.
Gastric emptying studies are performed with radionuclide-labeled meals. Emptying of solids and liquids can be assessed simultaneously when both phases are marked with different tracers. After ingestion of a labeled standard meal, gamma camera images of the stomach are obtained at 5- to 15-minute intervals for 1.5 to 2 hours. After correction for decay, the counts in the gastric area are plotted as the percentage of total counts at the start of the imaging. The resulting emptying curve can be compared with data obtained in normal volunteers. In general, normal subjects will empty 59% of a meal within 90 minutes. Although delayed gastric emptying is often associated with gastroesophageal reflux, in general delayed emptying does not correlate with a poorer clinical outcome after antireflux surgery, and it should not be considered a contraindication to surgical treatment.
Monitoring is performed over a complete circadian cycle with a pH electrode placed 5 cm below the manometrically located LES. The patient is fully ambulatory during the test and is encouraged to perform normal daily activity. The gastric pH profile is assessed separately for the meal, postprandial period, and fasting period. The latter is divided into the time spent upright and supine.
The interpretation of continuous gastric pH recordings is more difficult than that of esophageal pH recordings. This is because the gastric pH environment is determined by a complex interplay of acid secretion; mucus secretion; ingested food; swallowed saliva; regurgitated duodenal, pancreatic, and biliary secretions; and the effectiveness of the mixing and evacuation of the chyme. Using 24-hour gastric pH monitoring to evaluate the gastric secretory state is based on studies that have shown that a good correlation exists between increased basal acid output on standard gastric acid analysis, and a left shift on the frequency distribution graph of gastric pH recordings during the supine fasting period. The evaluation of gastric emptying by 24-hour gastric pH monitoring is based on studies demonstrating a good correlation between the emptying of a solid meal and the duration of the postprandial plateau and decline phase of the gastric pH record.
Using 24-hour gastric pH monitoring to evaluate duodenogastric reflux is based on the observation that reflux of alkaline duodenal juice into the stomach can alkalinize the gastric pH environment. The measurement is not straightforward because of the effect of meals, and reduction in acid secretion can result in changes in gastric pH that mimic alkaline reflux episodes. To overcome this problem, computerized measurements of the number and height of alkalinizing peaks, the baseline pH, the postprandial pH plateau, and the pattern of pH decline from the plateau can be used to identify the probability of duodenogastric reflux. The results are presented as an overall score that indicates the likelihood of pathologic duodenogastric reflux. Initial data indicate that this approach has a higher sensitivity and specificity for the diagnosis of pathologic duodenogastric reflux than scintigraphic methods do.
Combined 24-hour esophageal and gastric pH monitoring can identify excessive alkaline duodenogastric and alkaline gastroesophageal reflux in symptomatic patients. The combined tracings can often identify simultaneous gastric and esophageal alkalinization, suggesting a duodenal origin for the esophageal alkaline exposure (Fig. 25-25).
Figure 25-25.
A. Combined esophageal and gastric pH monitoring showing position of probes in relation to the lower esophageal sphincter. B. Combined ambulatory esophageal (upper tracing) and gastric (lower tracing) pH monitoring showing duodenogastric reflux (arrows) with propagation of the alkaline juice into the esophagus of a patient with complicated Barrett’s esophagus. The gastric tracing (lower) is taken from a probe lying 5 cm below the upper esophageal sphincter. The esophageal tracing (upper) is taken from a probe lying 5 cm above the lower esophageal sphincter. Note that in only a small proportion of time does duodenogastric reflux move the pH of the esophagus above the threshold of 7, causing the iceberg effect. (Reproduced with permission from DeMeester TR, Stein HJ, Fuchs KH: Physiologic diagnostic studies, in Zuidema GD, Orringer MB (eds): Shackelford’s Surgery of the Alimentary Tract, 3rd ed., Vol. I. Philadelphia: W.B. Saunders, 1991, p 123. Copyright Elsevier.)
GASTROESOPHAGEAL REFLUX DISEASE
GERD was not recognized as a significant clinical problem until the mid-1930s and was not identified as a precipitating cause for esophagitis until after World War II. In the early twenty-first century, it has grown to be a very common problem and now accounts for a majority of esophageal pathology. It is recognized as a chronic disease, and when medical therapy is required, it is often lifelong treatment. Recent efforts at the development of various endoscopic antireflux interventions, although innovative, have not been successful in consistently controlling gastroesophageal reflux. Antireflux surgery is an effective and long-term therapy and is the only treatment that is able to restore the gastroesophageal barrier. Despite the common prevalence of GERD, it can be one of the most challenging diagnostic and therapeutic problems in clinical medicine. A contributing factor to this is the lack of a universally accepted definition of the disease.
The most simplistic approach is to define the disease by its symptoms. However, symptoms thought to be indicative of GERD, such as heartburn or acid regurgitation, are very common in the general population and many individuals consider them to be normal and do not seek medical attention. Even when excessive, these symptoms are not specific for gastroesophageal reflux. They can be caused by other diseases such as achalasia, DES, esophageal carcinoma, pyloric stenosis, cholelithiasis, gastritis, gastric or duodenal ulcer, and coronary artery disease.
A thorough, structured evaluation of the patient’s symptoms is essential before any therapy, particularly any form of esophageal surgery. The presence and severity of both typical symptoms of heartburn, regurgitation, and dysphagia, and atypical symptoms of cough, hoarseness, chest pain, asthma, and aspiration should be discussed with the patient in detail. Many of these atypical symptoms may not be esophageal related and hence will not improve and may even worsen with antireflux surgery.
Heartburn is generally defined as a substernal burning-type discomfort, beginning in the epigastrium and radiating upward. It is often aggravated by meals, spicy or fatty foods, chocolate, alcohol, and coffee and can be worse in the supine position. It is commonly, although not universally, relieved by antacid or antisecretory medications. Epidemiologic studies have shown that heartburn occurs monthly in as many as 40% to 50% of the Western population. The occurrence of heartburn at night and its effect on quality of life have recently been highlighted by a Gallup poll conducted by the American Gastroenterologic Society (Table 25-4).
|
Regurgitation, the effortless return of acid or bitter gastric contents into the chest, pharynx, or mouth, is highly suggestive of foregut pathology. It is often particularly severe at night when supine or when bending over and can be secondary to either an incompetent or obstructed GEJ. With the later, as in achalasia, the regurgitant is often bland, as if food was put into a blender. When questioned, most patients can distinguish the two. It is the regurgitation of gastric contents that may result in associated pulmonary symptoms, including cough, hoarseness, asthma, and recurrent pneumonia. Bronchospasm can be precipitated by esophageal acidification and cough by either acid stimulation or distention of the esophagus.
Dysphagia, or difficulty swallowing, is a relatively nonspecific term but arguably the most specific symptom of foregut disease. It can be a sign of underlying malignancy and should be aggressively investigated until a diagnosis is established. Dysphagia refers to the sensation of difficulty in the passage of food from the mouth to the stomach and can be divided into oropharyngeal and esophageal etiologies. Oropharyngeal dysphagia is characterized by difficulty transferring food out of the mouth into the esophagus, nasal regurgitation, and/or aspiration. Esophageal dysphagia refers to the sensation of food sticking in the lower chest or epigastrium. This may or may not be accompanied by pain (odynophagia) that will be relieved by the passage of the bolus.
Chest pain, although commonly and appropriately attributed to cardiac disease, is frequently secondary to esophageal pathology as well. As early as 1982, DeMeester and associates showed that nearly 50% of patients with severe chest pain, normal cardiac function, and normal coronary arteriograms had positive 24-hour pH studies, implicating gastroesophageal reflux as the underlying etiology. Exercise-induced gastroesophageal reflux is well known to occur, and may result in exertional chest pain similar to angina. It can be quite difficult, if not impossible, to distinguish between the two etiologies, particularly on clinical grounds alone. Nevens and colleagues evaluated the ability of experienced cardiologists to differentiate pain of cardiac vs. esophageal origin. Of 248 patients initially seen by cardiologists, 185 were thought to have typical angina and 63 atypical chest pain. Forty eight (26%) of those thought to have classic angina had normal coronary angiograms and 16 of the 63 with atypical pain had abnormal angiogram. Thus, the cardiologists’ clinical impression was wrong 25% of the time. Finally, Pope and associates investigated the ultimate diagnosis in 10,689 patients presenting to an emergency room with acute chest pain. Approximately 17% were found to have acute ischemia, 6% stable angina, 21% other cardiac causes, and 55% had noncardiac causes. They concluded that the majority of people presenting to the emergency room with chest pain do not have an underlying cardiac etiology for their symptoms. Chest pain precipitated by meals, occurring at night while supine, nonradiating, responsive to antacid medication, or accompanied by other symptoms suggesting esophageal disease such as dysphagia or regurgitation should trigger the thought of possible esophageal origin. Further, the distinction between heartburn and chest pain is also difficult and largely dependent upon the individual patient. One person’s heartburn is another’s chest pain.
The precise mechanisms accounting for the generation of symptoms secondary to esophageal pathology remain unclear. Considerable insight has been acquired, however. Investigations into the effect of luminal content, esophageal distention and muscular function, neural pathways, and brain localization have provided a basic understanding of the stimuli responsible for symptom generation. It is also clear that the visceroneural pathways of the foregut are complexly intertwined with that of the tracheobronchial tree and heart. This fact accounts for the common overlap of clinical presentations with diverse disease processes in upper GI, cardiac, and pulmonary systems.
There is a high-pressure zone located at the esophagogastric junction in humans. Although this is typically referred to as the lower esophageal “sphincter,” there are no distinct anatomical landmarks which define its beginning and end. Architecturally speaking, there is a specialized thickening in this region that is made up of the collar sling musculature and the clasp fibers. The collar sling is located on the greater curvature side of the junction, and the clasp fibers are located on the lesser curvature side. These muscles remain in tonic opposition until the act of swallowing, whereupon receptive relaxation occurs allowing passage of a food bolus into the stomach. In addition, the LES will also open when the gastric fundus is distended with gas and liquid, thus resulting in an unfolding of the valve and enabling venting of gas (a belch). Whether physiologic or pathologic, the common denominator for most episodes of gastroesophageal reflux is the loss of the high-pressure zone and thus, a decrease in the resistance it imparts to the retrograde flow of gastric juice into the esophageal body. The primary cause of GERD is secondary to the permanent attenuation of the collar sling musculature, with a resultant opening of the gastric cardia and loss of the high-pressure zone as measured with esophageal manometry.
As defined by esophageal manometry, there are three characteristics of the LES that work in unison to maintain its barrier function. These characteristics include the resting LES pressure, its overall length, and the intra-abdominal length that is exposed to the positive pressure environment of the abdomen (Table 25-5). The resistance to gastroesophageal reflux is a function of both the resting LES pressure and length over which this pressure is exerted. Thus, as the sphincter becomes shorter, a higher pressure will be required in order to prevent a given amount of reflux (Fig. 25-26). Much like the neck of a balloon as it is inflated, as the stomach fills and distends, sphincter length decreases. Therefore, if the overall length of the sphincter is permanently short from repeated distention of the fundus secondary to large volume meals, then with minimal episodes of gastric distention and pressure, there will be insufficient sphincter length for the barrier to remain competent, and reflux will occur.
A third characteristic of the LES that impacts its ability to prevent reflux is its position about the diaphragm. It is important that a portion of the total length of the LES be exposed to the effects of an intra-abdominal pressure. That is, during periods of elevated intra-abdominal pressure, the resistance of the barrier would be overcome if pressure were not applied equally to both the LES and stomach simultaneously. Thus, in the presence of a hiatal hernia, the sphincter resides entirely within the chest cavity and cannot respond to an increase in intra-abdominal pressure because the pinch valve mechanism is lost and gastroesophageal reflux is more liable to occur.
Therefore, a permanently defective sphincter is defined by one or more of the following characteristics: An LES with a mean resting pressure of less than 6 mmHg, an overall sphincter length of <2 cm, and intra-abdominal sphincter length of <1 cm. Compared to normal subjects without GERD these values are below the 2.5 percentile for each parameter. The most common cause of a defective sphincter is an inadequate abdominal length.
Once the sphincter is permanently defective, this condition is irreversible, and although esophageal mucosal injury may be healed with antisecretory medication, reflux will continue to occur. Additionally, the presence of a defective LES may be associated with reduced esophageal body function and thus decrease clearance times of refluxed material. In addition, with the progressive loss of effective esophageal clearance the patient may be predisposed to severe mucosal injury, volume regurgitation, aspiration, and pulmonary failure. Reflux may occur in the face of a normal LES resting pressure. This condition is usually due to a functional problem of gastric emptying or excessive air swallowing. These conditions may lead to gastric distention, increased intra-gastric pressure, a resultant shortening or unfolding of the LES, and subsequent reflux. The mechanism by which gastric distention contributes to LES unfolding provides a mechanical explanation for “transient LES relaxation.” It is thought that with repeated gastric distention secondary to large meal volume or chronic air swallowing, there is repeated unfolding of the LES and subsequent attenuation of the collar sling musculature. It is at this point that the physiologic and normal mechanism of gastric venting is replaced with pathologic and severe postprandial reflux disease. In addition, patients with GERD will increase the frequency of swallowing in an effort to neutralize the refluxed acid with their saliva (pH 7.0). This phenomenon leads to increased air swallowing and further gastric distention thus, compounding the problem. Therefore GERD may have its origins in the stomach secondary to gastric distention due to overeating, and this may be further compounded by the ingestion of fatty meals, which result in delayed gastric emptying.
As the collar sling musculature and clasp fibers become attenuated with repeated gastric distention, the esophagogastric junction begins to assume an “upside down funnel” appearance, with progressive opening of the acute angle of His. This in turn may result in attenuation and stretching of the phrenoesophageal ligament, with subsequent enlargement of the hiatal opening and axial herniation. There is a high degree of correlation between reflux threshold and the degree of hiatal herniation (Fig. 25-27).
It is believed that the source of postprandial esophageal acid exposure results from a “pocket of acid” at the esophageal gastric junction that is unaffected by the buffering action of the meal. This same process is thought to occur in patients with endoscopy-negative dyspepsia and normal conventional esophageal pH monitoring at a location of 5 cm proximal to the upper boarder of the LES.
It is believed that GERD has its origins within the stomach. Distention of the fundus occurs because of overeating and delayed gastric emptying secondary to a high-fat diet. The resultant distention causes “unrolling” of the sphincter by the expanding fundus, and this subsequently exposes the squamous epithelium in the region of the distal LES to gastric juice. Repeated exposure results in inflammation and the development of columnar epithelium at the cardia. This is the initial step of the development of carditis and explains why in early disease esophagitis is mild and commonly limited to the very distal aspect of the esophagus. The patient attempts to compensate for this by increased swallowing, allowing the saliva to neutralize the refluxed gastric juice and thus, alleviate the discomfort induced by the reflux event. The increased swallowing results in aerophagia, bloating, and belching. This in turn creates a viscous cycle of increased gastric distention and thus further exposure and repetitive injury to the distal esophagus. The development of carditis explains the complaint of epigastric pain often experienced by patients with early reflux disease. Additionally this process can lead to a fibrotic mucosal ring located at the squamocolumnar junction, which is termed a “Schatzki ring,” and may result in dysphagia. This inflammatory process may extend into muscularis propria and thus result in a progressive loss in the length and pressure of the LES. This explanation for the pathophysiology of GERD is supported by the observation that severe esophagitis is almost always associated with a defective LES.
The complications of gastroesophageal reflux disease may result from the direct injurious effects of gastric fluid on the mucosa, larynx, or respiratory epithelium. Complications due to repetitive reflux are esophagitis, stricture, and BE; repetitive aspiration may lead to progressive pulmonary fibrosis. The severity of the complications is directly related to the prevalence of a structurally defective sphincter (Table 25-6). The observation that a structurally defective sphincter occurs in 42% of patients without complications (most of whom have one or two components failed) suggests that disease may be confined to the sphincter due to compensation by a vigorously contracting esophageal body. Eventually, all three components of the sphincter fail, allowing unrestricted reflux of gastric juice into the esophagus and overwhelming its normal clearance mechanisms. This leads to esophageal mucosal injury with progressive deterioration of esophageal contractility, as is commonly seen in patients with strictures and BE. The loss of esophageal clearance increases the potential for regurgitation into the pharynx with aspiration.
COMPLICATION | NO. | STRUCTURALLY NORMAL SPHINCTER (%) | STRUCTURALLY DEFECTIVE SPHINCTER (%) |
---|---|---|---|
None | 59 | 58 | 42 |
Erosive esophagitis | 47 | 23 | 77a |
Stricture | 19 | 11 | 89 |
Barrett’s esophagus | 25 | 0 | 100 |
Total | 150 |
The potential injurious components that reflux into the esophagus include gastric secretions such as acid and pepsin, as well as biliary and pancreatic secretions that regurgitate from the duodenum into the stomach. There is a considerable body of experimental evidence to indicate that maximal epithelial injury occurs during exposure to bile salts combined with acid and pepsin. These studies have shown that acid alone does minimal damage to the esophageal mucosa, but the combination of acid and pepsin is highly deleterious. Similarly, the reflux of duodenal juice alone does little damage to the mucosa, although the combination of duodenal juice and gastric acid is particularly noxious.
Experimental animal studies have shown that the reflux of duodenal contents into the esophagus enhances inflammation, increases the prevalence of Barrett’s esophagus, and results in the development of esophageal adenocarcinoma. The component of duodenal juice thought to be most damaging is bile acids. For bile acids to injure mucosal cells, it is necessary that they be both soluble and un-ionized, so that the un-ionized, nonpolar form may enter mucosal cells. Before the entry of bile into the GI tract, 98% of bile acids are conjugated with either taurine or glycine in a ratio of about 3:1. Conjugation increases the solubility and ionization of bile acids by lowering their pKa. At the normal duodenal pH of approximately 7, over 90% of bile salts are in solution and completely ionized. At pH ranges from 2 to 7, there is a mixture of the ionized salt and the lipophilic, nonionized acid. Acidification of bile to below pH 2 results in an irreversible bile acid precipitation. Consequently, under normal physiologic conditions, bile acids precipitate and are of minimal consequence when an acid gastric environment exists. On the other hand, in a more alkaline gastric environment, such as occurs with excessive duodenogastric reflux and after acid suppression therapy or vagotomy and partial or total gastrectomy, bile salts remain in solution, are partially dissociated, and when refluxed into the esophagus can cause severe mucosal injury by crossing the cell membrane and damaging the mitochondria.
Complications of gastroesophageal reflux such as esophagitis, stricture, and Barrett’s metaplasia occur in the presence of two predisposing factors: a mechanically defective LES and an increased esophageal exposure to fluid containing duodenal content which includes bile and pancreatic juice. The duodenal origin of esophageal contents in patients with an increased exposure to a pH >7 has previously been confirmed by esophageal aspiration studies (Fig. 25-28). Studies have clarified and expanded these observations by measuring esophageal bilirubin exposure over a 24-hour period as a marker for the presence of duodenal juice. Direct measurement of esophageal bilirubin exposure as a marker for duodenal juice has shown that 58% of patients with GERD have increased esophageal exposure to duodenal juice, and that this exposure occurs most commonly when the esophageal pH is between 4 and 7 (Fig. 25-29). These earlier studies have been confirmed by other studies which measure volume reflux using impedance technology.
Figure 25-28.
Sample bile acid concentration and esophageal pH plotted against time to obtain detailed profiles; in this case showing both significant bile acid (vertical bars) and acid (linear plot) reflux. (Reproduced with permission from Nehra D, Watt P, Pye JK, et al.: Automated oesophageal reflux sampler: A new device used to monitor bile acid reflux in patients with gastroesophageal reflux disease. J Med Engr Tech 21:1, 1997.)
Figure 25-29.
A. Prevalence of reflux types in 53 patients with gastroesophageal reflux disease. B. Esophageal luminal pH during bilirubin exposure. (Reproduced with permission from Kauer WK, Peters JH, DeMeester TR, et al.: Mixed reflux of gastric juice is more harmful to the esophagus than gastric juice alone: The need for surgical therapy reemphasized. Ann Surg. 222:525, 1995.)
The fact that the combination of refluxed gastric and duodenal juice is more noxious to the esophageal mucosa than gastric juice alone may explain the repeated observation that 25% of patients with reflux esophagitis develop recurrent and/or progressive mucosal damage, often despite medical therapy (Fig. 25-30). A potential reason is that acid suppression therapy is unable to consistently maintain the pH of refluxed gastric and duodenal juice above the range of 6. Lapses into pH ranges from 2 to 6 encourage the formation of undissociated, nonpolarized, soluble bile acids, which are capable of penetrating the cell wall and injuring mucosal cells. To ensure that bile acids remain completely ionized in their polarized form, and thus unable to penetrate the cell, requires that the pH of the refluxed material be maintained above 7, 24 hours a day, 7 days a week, for the patient’s lifetime. In practice, this would not only be impractical but likely impossible, unless very high doses of medications were used. The use of lesser doses would allow esophageal mucosal damage to occur while the patient was relatively asymptomatic. Antireflux operative procedures re-establish the barrier between stomach and esophagus, protecting the esophagus from damage in patients with mixed gastroesophageal reflux.
Figure 25-30.
Prevalence of abnormal esophageal bilirubin exposure in healthy subjects and in patients with gastroesophageal reflux disease with varied degrees of mucosal injury. (*P<.03 vs all other groups, **P <.03 vs. healthy subjects.) (Reproduced with permission from Kauer WK, Peters JH, DeMeester TR, et al.: Mixed reflux of gastric juice is more harmful to the esophagus than gastric juice alone: The need for surgical therapy reemphasized. Ann Surg. 222:525, 1995.)
If reflux of gastric juice is allowed to persist and sustained or repetitive esophageal injury occurs, two sequelae can result. First, a luminal stricture can develop from submucosal and eventually intramural fibrosis. Second, the tubular esophagus may become replaced with columnar epithelium. The columnar epithelium is resistant to acid and is associated with the alleviation of the complaint of heartburn. This columnar epithelium often becomes intestinalized, identified histologically by the presence of goblet cells. This specialized IM is currently required for the diagnosis of BE. Endoscopically, BE can be quiescent or associated with complications of esophagitis, stricture, Barrett’s ulceration, and dysplasia. The complications associated with BE may be due to the continuous irritation from refluxed duodenogastric juice. This continued injury is pH dependent and may be modified by medical therapy. The incidence of metaplastic Barrett’s epithelium becoming dysplastic and progressing to adenocarcinoma is approximately 0.2% to 0.5% per year.
An esophageal stricture can be associated with severe esophagitis or BE. In the latter situation, it occurs at the site of maximal inflammatory injury (i.e., the columnar-squamous epithelial interface). As the columnar epithelium advances into the area of inflammation, the inflammation extends higher into the proximal esophagus, and the site of the stricture moves progressively up the esophagus. Patients who have a stricture in the absence of Barrett’s esophagus should have the presence of gastroesophageal reflux documented before the presence of the stricture is ascribed to reflux esophagitis. In patients with normal acid exposure, the stricture may be due to cancer or a drug-induced chemical injury, the latter resulting from the lodgment of a capsule or tablet in the distal esophagus. In such patients, dilation usually corrects the problem of dysphagia. Heartburn, which may have occurred only because of the chemical injury, need not be treated. It is also possible for drug-induced injuries to occur in patients who have underlying esophagitis and a distal esophageal stricture secondary to gastroesophageal reflux. In this situation, a long, string-like stricture progressively develops as a result of repetitive caustic injury from capsule or tablet lodgment on top of an initial reflux stricture. These strictures are often resistant to dilation. The incidence of this problem has lessened since the introduction of proton pump inhibitor medication.
The condition whereby the tubular esophagus is lined with columnar epithelium rather than squamous epithelium was first described by Norman Barrett in 1950. He incorrectly believed it to be congenital in origin. It is now realized that it is an acquired abnormality, occurs in 10% to 15% of patients with GERD, and represents the end stage of the natural history of this disease. It is also distinctly different from the congenital condition in which islands of gastric fundic epithelium are found in the upper half of the esophagus.
The definition of BE has evolved considerably over the past decade. Traditionally, BE was identified by the presence of columnar mucosa extending at least 3 cm into the esophagus. It is now recognized that the specialized, intestinal-type epithelium found in the Barrett’s mucosa is the only tissue predisposed to malignant degeneration. Consequently, the diagnosis of BE is presently made given any length of endoscopically identifiable columnar mucosa that proves, on biopsy, to show IM. Although long segments of columnar mucosa without IM do occur, they are uncommon and might be congenital in origin.
The hallmark of IM is the presence of intestinal goblet cells. There is a high prevalence of biopsy-demonstrated IM at the cardia, on the gastric side of the squamocolumnar junction, in the absence of endoscopic evidence of a CLE. Evidence is accumulating that these patches of what appears to be Barrett’s in the cardia have a similar malignant potential as in the longer segments, and are precursors for carcinoma of the cardia.
The long-term relief of symptoms remains the primary reason for performing antireflux surgery in patients with BE. Healing of esophageal mucosal injury and the prevention of disease progression are important secondary goals. In this regard, patients with BE are no different than the broader population of patients with gastroesophageal reflux. They should be considered for antireflux surgery when patient data suggest severe disease or predict the need for long-term medical management. Most patients with BE are symptomatic. Although it has been argued that some patients with BE may not have symptoms, careful history taking will reveal the presence of symptoms in most, if not all, patients.
Patients with BE have a spectrum of disease ranging from visually identifiable but short segments, to long segments of classic BE. In general, however, they represent a relatively severe stage of gastroesophageal reflux, usually with markedly increased esophageal acid exposure, deficient LES characteristics, poor esophageal body function, and a high prevalence of duodenogastroesophageal reflux. Gastric hypersecretion occurs in 44% of patients. Most will require long-term PPI therapy for relief of symptoms and control of coexistent esophageal mucosal injury. Given such profound deficits in esophageal physiology, antireflux surgery is an excellent means of long-term control of reflux symptoms for most patients with BE.
The typical complications in BE include ulceration in the columnar-lined segment, stricture formation, and a dysplasia-cancer sequence. Barrett’s ulceration is unlike the erosive ulceration of reflux esophagitis in that it more closely resembles peptic ulceration in the stomach or duodenum, and has the same propensity to bleed, penetrate, or perforate. Fortunately this complication occurs very rarely. The strictures found in BE occur at the squamocolumnar junction, and are typically higher than peptic strictures in the absence of BE. Ulceration and stricture in association with BE were commonly reported before 1975, but, with the advent of potent acid suppression medication, they have become less common. In contrast, the complication of adenocarcinoma developing in Barrett’s mucosa has become more common. Adenocarcinoma developing in Barrett’s mucosa was considered a rare tumor before 1975. Today, it occurs at approximately 0.2% to 0.5% per year of follow-up, which represents a risk 40 times that of the general population. Most, if not all, cases of adenocarcinoma of the esophagus arise in Barrett’s epithelium (Fig. 25-31). About one-third of all patients with BE present with malignancy.
Figure 25-31.
Photomicrographs. A. Barrett’s epithelium with severe dysplasia. (×200.) Note nuclear irregularity, stratification, and loss of polarity. B. Barrett’s epithelium with intramucosal carcinoma. (×66.) Note malignant cells in the mucosa (upper arrow), but not invading the muscularis mucosae (bottomarrow). (Reproduced with permission from DeMeester TR, Stein HJ, Fuchs KH: Physiologic diagnostic studies, in Zuidema GD, Orringer MB (eds): Shackelford’s Surgery of the Alimentary Tract, 3rd ed, Vol. I. Philadelphia: W.B. Saunders, 1991, p 113. Copyright Elsevier.)
The long-term risk of progression to dysplasia and adenocarcinoma, although not the driving force behind the decision to perform antireflux surgery, is a significant concern for both patient and physician. Although to date, there have been no prospective randomized studies documenting that antireflux surgery has an effect on the risk of progression to dysplasia and carcinoma, complete control of reflux of gastric juice into the esophagus is clearly a desirable goal.
A significant proportion of patients with GERD will have associated respiratory symptoms. These patients may have laryngopharyngeal reflux-type symptoms, adult-onset asthma, or even idiopathic pulmonary fibrosis. These symptoms and organ injury may occur in isolation or in conjunction with typical reflux symptoms such as heartburn and regurgitation. Several studies have demonstrated that up to 50% of patients with asthma have either endoscopically evident esophagitis or abnormal distal esophageal acid exposure. These findings support a causal relationship between GERD and aerodigestive symptoms and complications in a proportion of patients.
There are two mechanisms that have been proposed as the cause of reflux-induced respiratory symptoms. The reflux theory suggests that these symptoms are the direct result of laryngopharyngeal exposure and aspiration of gastric contents. The reflex theory suggests that the vagal-mediated afferent fibers result in bronchoconstriction during episodes of distal esophageal acidification. The evidence supporting a mechanism of direct exposure to the aerodigestive system is based in clinical studies that have documented a strong correlation between idiopathic pulmonary fibrosis and hiatal hernia. In addition, the presence of GERD was demonstrated to be highly associated with several pulmonary diseases in a recent Department of Veteran Affairs multivariate analysis. Next, with ambulatory pH testing, acid exposure within the proximal esophagus is more frequently identified in patients with gastroesophageal reflux and respiratory symptoms than in patients who have gastroesophageal reflux symptoms alone. These findings are supported by scintigraphic studies, which have demonstrated aspiration of ingested radioisotope in patients with both gastroesophageal reflux and pulmonary symptoms. In animal studies, tracheal instillation of acid has been demonstrated to profoundly increase airway resistance. Finally, in patients who have undergone multichannel intraluminal impedance testing with a catheter configured to detect laryngopharyngeal reflux, a correlation between proximal fluid movement and laryngopharyngeal symptoms, such as cough, can be demonstrated.
The reflex mechanism is supported by the bronchoconstriction that occurs with the infusion of acid into the distal esophagus. There is a shared embryologic origin of the tracheoesophageal tract and vagus nerve, and this reflex is thought to be an afferent fiber–mediated reflex that protects the aerodigestive system from the aspiration of refluxate. In patients with respiratory symptoms and documented gastroesophageal reflux without proximal esophageal acid exposure, pulmonary symptoms will often times significantly improve or completely resolve after undergoing laparoscopic fundoplication. It is likely that both of the proposed mechanisms work simultaneously to cause these symptoms in the face of GERD.
The most difficult clinical challenge in formulating a treatment plan for reflux-associated respiratory symptoms resides in establishing the diagnosis. Although the diagnosis made be straightforward in patients with predominately typical reflux symptoms and secondary respiratory complaints, a substantial number of patients will have respiratory symptoms that dominate the clinical scenario. Typical gastroesophageal reflux symptoms, such as heartburn and regurgitation, may often be completely absent only to be uncovered with objective esophageal physiology testing. Traditionally, the diagnosis of reflux-induced respiratory injury is established using ambulatory dual probe pH monitoring, with one probe positioned within the distal esophagus and the other at a proximal location. Proximal probe positioning has included multiple locations such as the trachea, pharynx, and proximal esophagus. Although ambulatory esophageal pH monitoring allows a direct correlation between esophageal acidification and respiratory symptoms, sensitivity of this testing modality is poor, and the temporal relationship between laryngeal or pulmonary symptoms and reflux events is complex. In addition, as the refluxed gastric fluid travels proximally, it may be neutralized by saliva and therefore go undetected with pH monitoring. Impedance testing may also be used to detect the movement of fluid throughout the entire esophageal column regardless of pH content.
Once the diagnosis is established, treatment may be initiated with either PPI therapy or antireflux surgery. A trial of high-dose PPI therapy may help establish the facts that reflux is partly or completely responsible for the respiratory symptoms. It is important to note that the persistence of symptoms in the face of aggressive PPI treatment does not necessarily rule out reflux as a possible cofactor or sole etiology.
Although there is probably some elements of a placebo effect, relief of respiratory symptoms can be anticipated in up to 50% of patients with reflux-induced asthma treated with antisecretory medications. However, when examined objectively, <15% of patients can be expected to have improvement in their pulmonary function with medical therapy. In properly selected patients, antireflux surgery improves respiratory symptoms in nearly 90% of children and 70% of adults with asthma and reflux disease. Improvements in pulmonary function can be demonstrated in around 30% of patients. Uncontrolled studies of the two forms of therapy (PPI and surgery) and the evidence from the two randomized controlled trials of medical vs. surgical therapy indicate that surgical valve reconstruction is the most effective therapy for reflux-induced asthma. The superiority of the surgery over PPI is most noticeable in the supine position, which corresponds with the nadir of PPI blood levels and resultant acid breakthrough and is the time in the circadian cycle when asthma symptoms are at their worst.
In asthmatic patients with an esophageal motility disorder, performing an antireflux operation will not prevent the regurgitation and possible aspiration of swallowed liquid or food “upstream” to the valve reconstruction. It is critical that esophageal body function be considered prior to surgical intervention in this patient population.
With the widespread availability of over-the-counter antisecretory medications, most patients with mild or moderate symptoms will carry self-medication. When initially identified with mild symptoms of uncomplicated GERD, patients can be placed on 12 weeks of simple antacids before diagnostic testing is initiated. This approach may successfully and completely resolve the symptoms. Patients should be counseled to elevate the head of the bed; avoid tight-fitting clothing; eat small, frequent meals; avoid eating the nighttime meal immediately prior to bedtime; and avoid alcohol, coffee, chocolate, and peppermint, which are known to reduce resting LES pressure and may aggravate symptoms.
Used in combination with simple antacids, alginic acid may augment the relief of symptoms by creating a physical barrier to reflux, as well as by acid reduction. Alginic acid reacts with sodium bicarbonate in the presence of saliva to form a highly viscous solution that floats like a raft on the surface of the gastric contents. When reflux occurs, this protective layer is refluxed into the esophagus, and acts as a protective barrier against the noxious gastric contents. Medications to promote gastric emptying, such as metoclopramide or domperidone, are beneficial in early disease, but of little value in more severe disease.
In patients with persistent symptoms, the mainstay of medical therapy is acid suppression. High-dosage regimens of hydrogen potassium PPIs, such as omeprazole (up to 40 mg/d), can reduce gastric acidity by as much as 80% to 90%. This usually heals mild esophagitis. In severe esophagitis, healing may occur in only one-half of the patients. In patients who reflux a combination of gastric and duodenal juice, acid-suppression therapy may give relief of symptoms, while still allowing mixed reflux to occur. This can allow persistent mucosal damage in an asymptomatic patient. Unfortunately, within 6 months of discontinuation of any form of medical therapy for GERD, 80% of patients have a recurrence of symptoms.
Once initiated, most patients with GERD will require lifelong treatment with PPIs, both to relieve symptoms and control any coexistent esophagitis or stricture. Although control of symptoms has historically served as the endpoint of therapy, the wisdom of this approach has recently been questioned, particularly in patients with BE. Evidence suggesting that reflux control may prevent the development of adenocarcinoma and lead to regression of dysplastic and nondysplastic Barrett’s segments has led many to consider control of reflux, and not symptom control, a better therapeutic endpoint. However, this hypothesis remains controversial. It should be noted that complete control of reflux using PPIs can be difficult, as has been highlighted by studies of acid breakthrough while on PPI therapy, and of persistent reflux following antireflux surgery. Castell, Triadafilopoulos, and others have shown that 40% to 80% of patients with BE continue to have abnormal esophageal acid exposure despite up to 20 mg twice daily of PPIs. Ablation trials have shown that mean doses of 56 mg of omeprazole were necessary to normalize 24-hour esophageal pH studies. It is likely that antireflux surgery results in more reproducible and reliable elimination of reflux of both acid and duodenal contents, although long-term outcome studies suggest that as many as 25% of postfundoplication patients will have persistent pathologic esophageal acid exposure confirmed by positive 24-hour pH studies.
Traditionally a stepwise approach is used for the treatment of GERD. First-line therapy entails antisecretory medication, usually PPIs, in most patients. Failure of medication, or the immediate return of symptoms after stopping treatment, suggests either that the patient may have relatively severe disease or a non-GERD cause for his or her symptoms. Endoscopic examination at this stage of the patient’s evaluation is recommended and will provide the opportunity to assess the degree of mucosal injury and presence of BE. Treatment options for these patients entails either long term PPI use vs. antireflux surgery. Laparoscopic antireflux surgery in these patients achieves long-term control of symptoms in 85% to 90%. The measurement of esophageal acid exposure via 24-hour pH should be undertaken when patients are considered for surgery. The status of the LES and esophageal body function with esophageal manometry should also be performed at this stage. These studies will serve to establish the diagnosis and assess esophageal body dysfunction.
Studies of the natural history of GERD indicate that most patients have a relatively benign form of the disease that is responsive to lifestyle changes and dietary and medical therapy, and do not need surgical treatment. Approximately 25% to 50% of the patients with GERD have persistent or progressive disease, and it is this patient population that is best suited to surgical therapy. In the past, the presence of esophagitis and a structurally defective LES were the primary indications for surgical treatment, and many internists and surgeons were reluctant to recommend operative procedures in their absence. However, one should not be deterred from considering antireflux surgery in a symptomatic patient with or without esophagitis or a defective sphincter, provided the disease process has been objectively documented by 24-hour pH monitoring. This is particularly true in patients who have become dependent upon therapy with PPIs, or require increasing doses to control their symptoms. It is important to note that a good response to medical therapy in this group of patients predicts an excellent outcome following antireflux surgery.
In general, the key indications for antireflux surgery are (a) objectively proven gastroesophageal reflux disease, and (b) typical symptoms of gastroesophageal reflux disease (heartburn and/or regurgitation) despite adequate medical management, or (c) a younger patient unwilling to take lifelong medication. In addition, a structurally defective LES can also predict which patients are more likely to fail with medical therapy. Patients with normal sphincter pressures tend to remain well controlled with medical therapy, whereas patients with a structurally defective LES may not respond as well to medical therapy, and often develop recurrent symptoms within 1 to 2 years of beginning therapy. Such patients should be considered for an antireflux operation, regardless of the presence or absence of endoscopic esophagitis.
Young patients with documented reflux disease with or without a defective LES are also excellent candidates for antireflux surgery. They usually will require long-term medical therapy for control of their symptoms, and some will go on to develop complications of the disease. An analysis of the cost of therapy based on data from the Veterans Administration Cooperative trial indicates that surgery has a cost advantage over medical therapy in patients <49 years of age.
Severe endoscopic esophagitis in a symptomatic patient with a structurally defective LES is also an indication for early surgical therapy. These patients are prone to breakthrough of their symptoms while receiving medical therapy. Symptoms and mucosal injury can be controlled in such patients, but careful monitoring is required, and increasing dosages of PPIs are necessary. In everyday clinical practice, however, such treatment can be both difficult and impractical, and, in such cases, antireflux surgery can be considered early, especially if PPI therapy is problematic.
The development of a stricture in a patient represents a failure of medical therapy, and is also an indication for a surgical antireflux procedure. In addition, strictures are often associated with a structurally defective sphincter and loss of esophageal contractility. Before proceeding with surgical treatment, malignancy and a drug-related etiology of the stricture should be excluded, and the stricture progressively dilated up to a 50 to 60F bougie. When the stricture is fully dilated, the relief of dysphagia is evaluated and esophageal manometry is performed to determine the adequacy of peristalsis in the distal esophagus. If dysphagia is relieved and the amplitude of esophageal contractions is adequate, an antireflux procedure should be performed; if there is a global loss of esophageal contractility, caution should be exercised in performing an antireflux procedure with a complete fundoplication, and a partial fundoplication should be considered.
Barrett’s CLE is commonly associated with a severe structural defect of the LES and often poor contractility of the esophageal body. Patients with BE are at risk of the development of an adenocarcinoma. Whilst surgeons would like to think that an antireflux procedure can reduce the risk of progression to cancer, the evidence supporting this is relatively weak, and for now Barrett’s esophagus should be considered to be evidence that the patient has gastroesophageal reflux, and progression to antireflux surgery is indicated for the treatment of reflux symptoms, not cancer progression. If, however, high grade dysplasia or intramucosal carcinoma is found on mucosal biopsy specimens, treatment should then be directed at the BE and the lesion, using either evaluation endoscopic ablation, endoscopic resection or esophageal resection.
The majority of patients requiring treatment for reflux have a relatively mild form of disease and will respond to antisecretory medications. Patients with more severe forms of disease, particularly those who develop persistent or progressive disease, should be considered for definitive therapy. Laparoscopic fundoplication will provide a long-term cure in the majority of these patients, with minimal discomfort and an early return to normal activity.
Before proceeding with an antireflux operation, several factors should be evaluated. The clinical symptoms should be consistent with the diagnosis of gastroesophageal reflux. Patients presenting with the typical symptoms of heartburn and/or regurgitation which have responded, at least partly, to PPI therapy, will generally do well following surgery, whereas patients with atypical symptoms have a less predictable response. Reflux should also be objectively confirmed by either the presence of ulcerative esophagitis or an abnormal 24 hour pH study.
The propulsive force of the body of the esophagus should be evaluated by esophageal manometry to determine if it has sufficient power to propel a bolus of food through a newly reconstructed valve. Patients with normal peristaltic contractions can be considered for a 360° Nissen fundoplication or a partial fundoplication, depending on patient and surgeon preferences. When peristalsis is absent a partial fundoplication is probably the procedure of choice, but only if achalasia has been ruled out.
Hiatal anatomy should also be assessed. In patients with smaller hiatal hernias endoscopy evaluation usually provides sufficient information. However, when patients present with a very large hiatus hernia or for revision surgery after previous antireflux surgery, contrast radiology provides better anatomical information. The concept of anatomic shortening of the esophagus is controversial, with divergent opinions held about how common this problem is. Believers claim that anatomic shortening of the esophagus compromises the ability of the surgeon to perform an adequate repair without tension, and that this can lead to an increased incidence of breakdown or thoracic displacement of the repair. Some of those that hold this view claim that esophageal shortening is present when a barium swallow X-ray identifies a sliding hiatal hernia that will not reduce in the upright position, or that measures more than 5 cm in length at endoscopy. When identified these surgeons usually undertake add a gastroplasty to the antireflux procedure. Others claim that esophageal shortening is overdiagnosed and rarely seen, and that the morbidity of adding a gastroplasty outweighs any benefits. These surgeons would recommend a standard antireflux procedure in all patients undergoing primary surgery.
The primary goal of antireflux surgery is to safely create a new antireflux valve at the gastroesophageal junction, while preserving the patient’s ability to swallow normally and to belch to relieve gaseous distention. Regardless of the choice of the procedure, this goal can be achieved if attention is paid to some basic principles when reconstructing the antireflux mechanism. First, the operation should create a flap valve which prevents regurgitation of gastric contents into the esophagus. This will result in an increase in the pressure of the distal esophageal sphincter region. Following a Nissen fundoplication the expected increase is to a level twice the resting gastric pressure (i.e., 12 mmHg for a gastric pressure of 6 mmHg). The extent of the pressure rise is often less following a partial fundoplication, although with all types of fundoplication the length of the reconstructed valve should be at least 3 cm. This not only augments sphincter characteristics in patients in whom they are reduced before surgery, but prevents unfolding of a normal sphincter in response to gastric distention (Fig. 25-32). Preoperative and postoperative esophageal manometry measurements have shown that the resting sphincter pressure and the overall sphincter length can be surgically augmented over preoperative values, and that the change in the former is a function of the degree of gastric wrap around the esophagus (Fig. 25-33). However, the aim of any fundoplication is to create a loose wrap, and to maintain the position of the gastric fundus close to the distal intra-abdominal esophagus, in a flap valve arrangement. The efficacy of this relies on the close relationship between the fundus and the esophagus, not the “tightness” of the wrap.
Figure 25-33.
The relationship between the augmentation of sphincter pressure over preoperative pressure (ΔP) and the degree of gastric fundic wrap in three different antireflux procedures. (Reproduced with permission from O’Sullivan GC, et al.: Interaction of lower esophageal pressure and length of sphincter in the abdomen as detriments of gastroesophageal competence. Am J Surg. 143:43, 1982. Copyright Elsevier.)
Second, the operation should place an adequate length of the distal esophageal sphincter in the positive-pressure environment of the abdomen by a method that ensures its response to changes in intra-abdominal pressure. The permanent restoration of 2 or more cm of abdominal esophagus ensures the preservation of the relationship between the fundus and the esophagus. All of the popular antireflux procedures increase the length of the sphincter exposed to abdominal pressure by an average of at least 1 cm.
Third, the operation should allow the reconstructed cardia to relax on deglutition. In normal swallowing, a vagally mediated relaxation of the distal esophageal sphincter and the gastric fundus occurs. The relaxation lasts for approximately 10 seconds and is followed by a rapid recovery to the former tonicity. To ensure relaxation of the sphincter, three factors are important: (a) Only the fundus of the stomach should be used to buttress the sphincter, because it is known to relax in concert with the sphincter; (b) the gastric wrap should be properly placed around the sphincter and not incorporate a portion of the stomach or be placed around the stomach itself, because the body of the stomach does not relax with swallowing; and (c) damage to the vagal nerves during dissection of the thoracic esophagus should be avoided because it may result in failure of the sphincter to relax.
Fourth, the fundoplication should not increase the resistance of the relaxed sphincter to a level that exceeds the peristaltic power of the body of the esophagus. The resistance of the relaxed sphincter depends on the degree, length, and diameter of the gastric fundic wrap, and on the variation in intra-abdominal pressure. A 360° gastric wrap should be no longer than 2 cm and constructed over a large (50 to 60F) bougie. This will ensure that the relaxed sphincter will have an adequate diameter with minimal resistance. A bougie is not necessary when constructing a partial wrap.
Fifth, the operation should ensure that the fundoplication can be placed in the abdomen without undue tension, and maintained there by approximating the crura of the diaphragm above the repair. Leaving the fundoplication in the thorax converts a sliding hernia into a PEH, with all the complications associated with that condition. Maintaining the repair in the abdomen under tension predisposes to an increased incidence of recurrence. How common this problem is encountered is disputed, with some surgeons advocating lengthening the esophagus by gastroplasty and constructing a partial fundoplication, and others claiming that this issue is now rarely encountered.
A laparoscopic approach is now used routinely in all patients undergoing primary antireflux surgery. Some surgeons advocate the use of a single antireflux procedure for all patients, whereas others advocate a tailored approach. Advocates of the laparoscopic Nissen fundoplication as the procedure of choice for a primary antireflux repair would generally apply this procedure in all patients with normal or near normal esophageal motility, and reserve a partial fundoplication for use in individuals with poor esophageal body motility. Others, based on the good longer term outcomes now reported following partial fundoplication procedures, advocate the routine application of a partial fundoplication procedure, thereby avoiding any concerns about constructing a fundoplication in individuals with poor esophageal motility.
Experience and randomized studies have shown that both the Nissen fundoplication and various partial fundoplication procedures are all effective and durable antireflux repairs, and generate an excellent outcome in approximately 90% of patients at longer term follow-up.
The most common antireflux procedure is the Nissen fundoplication. In the past this procedure has been performed through an open abdominal or a chest incision, but with the development of laparoscopic approaches primary antireflux surgery is now routinely undertaken using the laparoscope. Rudolph Nissen described this procedure as a 360° fundoplication around the lower esophagus for a distance of 4 to 5 cm, without division of the short gastric blood vessels. Although this provided good control of reflux, it was associated with a number of side effects that have encouraged modifications of the procedure as originally described. These include using only the gastric fundus to envelop the esophagus in a fashion analogous to a Witzel jejunostomy, sizing the fundoplication with a large (50 to 60F) bougie, limiting the length of the fundoplication to 1 to 2 cm, and dividing the short gastric vessels. The essential elements necessary for the performance of a transabdominal fundoplication are common to both the laparoscopic and open procedures and include the following:
Hiatal dissection and preservation of both vagi along their entire length
Circumferential esophageal mobilization
Hiatal closure, usually posterior to the esophagus
Creation of a short and floppy fundoplication over an esophageal dilator
In addition, many surgeons also routinely divide the short gastric blood vessels, although this step is not universally applied, and the results of several randomized trials have failed to show that this step yields any benefit.
The laparoscopic approach to fundoplication has now replaced the open abdominal Nissen fundoplication as the procedure of choice. Five ports are usually used (Fig. 25-34), and dissection is begun by incising the gastrohepatic omentum above and below the hepatic branch of the anterior vagus nerve, which is usually preserved. The circumference of the diaphragmatic hiatus is dissected and the esophagus is mobilized by careful dissection of the anterior and posterior soft tissues within the hiatus. The esophagus is held anterior and to the left and the hiatal pillars are approximated with interrupted non-absorbable sutures, starting posteriorly and working anteriorly. A tension-free fundoplication should be constructed. This can usually be achieved either with or without division of the short gastric blood vessels, according to surgeon preference. If the vessels are divided, the upper one third of the greater curvature is mobilized by sequentially dissecting and divided these vessels, commencing distally and working proximally. Following complete fundal mobilization, the posterior wall of the fundus is brought behind the esophagus to the right side, and the anterior wall of the fundus is brought anterior to the esophagus. The fundic lips are manipulated to allow the fundus to envelop the esophagus without twisting. A 50 to 60F bougie is passed to properly size the fundoplication, and it is sutured using non-absorbable sutures. Some surgeons use a single U-stitch of 2-0 polypropylene buttressed with felt pledgets (Fig. 25-35), and others use 2-4 interrupted sutures.
Figure 25-34.
Patient positioning and trocar placement for laparoscopic antireflux surgery. The patient is placed with the head elevated approximately 30° in the modified lithotomy position. The surgeon stands between the patient’s legs, and the procedure is completed using five abdominal access ports.
Figure 25-35.
A. Laparoscopic Nissen fundoplication is performed with a five-trocar technique. B. The liver retractor is affixed to a mechanical arm to hold it in place throughout the operation. C. After division of the gastrohepatic omentum above the hepatic branch of the vagus (pars flaccida), the surgeon places a blunt atraumatic grasper beneath the phrenoesophageal ligament. D. After completion of the crural closure, an atraumatic grasper is placed right to left behind the gastroesophageal junction. The grasper is withdrawn, pulling the posterior aspect of the gastric fundus behind the esophagus. E. Once the suture positions are chosen, the first stitch (2-0 silk, 20 cm long) is introduced through the 10-mm trocar, and the needle is passed first through the left limb of the fundus, then the esophagus (2.5 cm above the gastroesophageal junction), then through the right limb of the fundus. F. Final position of the fundoplication. (Illustration continued on next page.)
Partial fundoplications were developed as an alternative to the Nissen procedure in an attempt to minimize the risk of postfundoplication side effects, such as dysphagia, inability to belch, and flatulence. The commonest approach has been a posterior partial or Toupet fundoplication. Some surgeons use this type of procedure for all patients presenting for antireflux surgery, whereas others apply a tailored approach in which a partial fundoplication is constructed in patients with impaired esophageal motility, in which the propulsive force of the esophagus is thought to be insufficient to overcome the outflow obstruction of a complete fundoplication. The Toupet posterior partial fundoplication consists of a 270° gastric fundoplication around the distal 4 cm of esophagus (Fig. 25-36). It is usually stabilized by anchoring the wrap posteriorly to the hiatal rim.
An alternative approach to partial fundoplication is to construct an anterior partial fundoplication. Following posterior hiatal repair, the anterior fundus is rolled over the front of the esophagus and sutured to the hiatal rim and the esophageal wall. Division of the short gastric vessels is never needed when constructing this type of fundoplication. Various degrees of anterior partial fundoplication have been described—90°, 120°, 180°. The anterior 180° partial fundoplication (Fig. 25-37) provides a more robust fundoplication and achieves an excellent longer term outcome in approximately 90% of patients at follow-up of at least 10 years. With this procedure the fundus and esophagus are sutured to the right side of the hiatal rim to create a flap valve at the gastroesophageal junction, and to stabilize a 3-4 cm length of intra-abdominal esophagus.
Figure 25-37.
Completed laparoscopic anterior 1800 partial fundoplication. The fundoplication fully covers the anterior surface of the esophagus, and is stabilized by suturing the fundus to the right side of the esophagus, and to the right hiatal pillar. Unlike the Nissen procedure, the fundus is not pulled behind the esophagus.
When a shortened esophagus is encountered, many surgeons choose to add an esophageal lengthening procedure before fundoplication, to reduce the tension on the gastro-esophageal junction, believing this will minimize the risk of failure due to postoperative hiatus hernia. The commonest approach to this is the Collis gastroplasty. This entails using a stapler to divide the cardia and upper stomach, parallel to the lesser curvature of the stomach, thereby creating a gastric tube in continuity with the esophagus, and effectively lengthening the esophagus by several centimeters. Laparoscopic techniques for Collis gastroplasty have been described (Fig. 25-38). Following gastroplasty a fundoplication is constructed, with the highest suture is placed on the native esophagus when constructing a Nissen fundoplication.Not all surgeons choose to undertake a Collis procedure, however, as there is controversy about the actual incidence of the shortened esophagus and widely divergent views are held about how often this problem is encountered. In addition, some surgeons have questioned the wisdom of creating an amotile tube of gastric wall, which can secrete acid, and then placing a Nissen fundoplication below this.
Figure 25-38.
A. After removal of the fat pad and release of tension on the Penrose drain, the gastroesophageal junction (GES) retracts to the level of the hiatus. The interior end of the staple line is marked 2/5 cm below the angle of His. B. The first horizontal firing of the stapler occurs by maximally articulating the stapler to the left, aiming toward the previously marked spot adjacent to the dilator. C. The vertical staple line is created by a single firing of the GIA placed parallel and flush against the 48F dilator. D. The highest Nissen fundoplication suture is placed on the native esophagus, and the second suture tucks in the apex of the staple line.
Studies of long-term outcome following both open and laparoscopic fundoplication document the ability of laparoscopic fundoplication to relieve typical reflux symptoms (heartburn, regurgitation, and dysphagia) in more than 90% of patients at follow-up intervals averaging 2 to 3 years and 80% to 90% of patients 5 years or more following surgery. This includes evidence-based reviews of antireflux surgery, prospective randomized trials comparing antireflux surgery to PPI therapy and open to laparoscopic fundoplication and analysis of U.S. national trends in use and outcomes. Postoperative pH studies indicate that more than 90% of patients will normalize their pH tracings. The results of laparoscopic fundoplication compare favorably with those of the “modern” era of open fundoplication. They also indicate the less predictable outcome of atypical reflux symptoms (cough, asthma, laryngitis) after surgery, being relieved in only two-thirds of patients.
The goal of surgical treatment for GERD is to relieve the symptoms of reflux by re-establishing the gastroesophageal barrier. The challenge is to accomplish this without inducing dysphagia or other untoward side effects. Dysphagia, existing before surgery, usually improves following laparoscopic fundoplication. Temporary dysphagia is common after surgery and generally resolves within 3 months, but can take up to 12 months in some individuals, and dysphagia sufficient to require ongoing dietary modification persists in up to 5% of individuals following Nissen fundoplication. Other side effects common to antireflux surgery include the inability to belch and vomit and increased flatulence. Most patients cannot vomit through an intact wrap, though this is rarely clinically relevant. Most patients are unable to belch gas from the stomach in the first 3 to 6 months after fundoplication, but 80%-90% regain the ability to belch normally beyond the first 12 months follow-up. Hyperflatulence is a common and noticeable problem, likely related to increased air swallowing that is present in most patients with reflux disease, aggravated by the inability to belch in some patients.
Originally, Nissen’s description of a total fundoplication entailed a 360° fundoplication during which the short gastric blood vessels were left intact. However, with reports of troublesome postoperative dysphagia division of these vessels, to achieve full fundal mobilization and thereby ensure a loose fundoplication, was promoted and has entered common practice. The evidence supporting dividing these vessels has been based on the outcomes from uncontrolled case series of patients undergoing Nissen fundoplication either with vs. without division of the short gastric vessels. However, the results from these studies have been conflicting with different proponents reporting good results irrespective of whether these vessels have been divided or not. To address this issue 6 randomized trials which enrolled a total of 438 patients have been reported. None of these trials demonstrated any differences for the postoperative dysphagia or recurrent gastro-esophageal reflux. However, in the 3 largest of the 6 trials an increased incidence of flatulence and bloating symptoms, as well as greater difficulty with belching, was seen in patients in whom the short gastric vessels were divided.
A recent meta-analysis from Engstrom et al, generated by combining the raw data from Australian and Swedish trials, evaluated a larger cohort of 201 patients, with 12 years follow-up in 170, and also confirmed equivalent reflux control, but more abdominal bloating after division of the short gastric vessels. Overall, these trials fail to support the belief that dividing the short gastric vessels improves any outcome following Nissen fundoplication. The trials actually suggest that dividing the vessels increases the complexity of the procedure, and leads to a poorer outcome due to the increase in bloating symptoms.
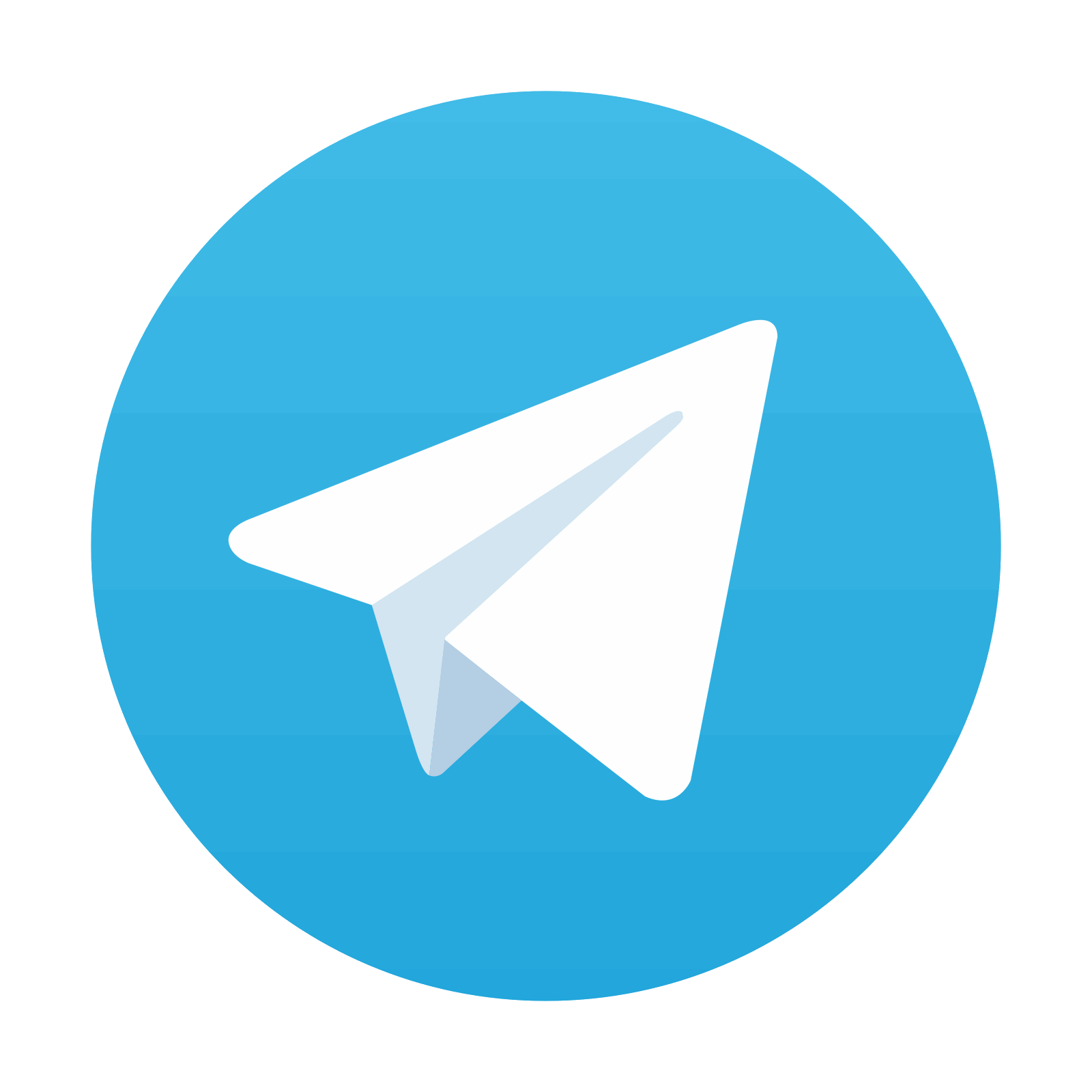
Stay updated, free articles. Join our Telegram channel

Full access? Get Clinical Tree
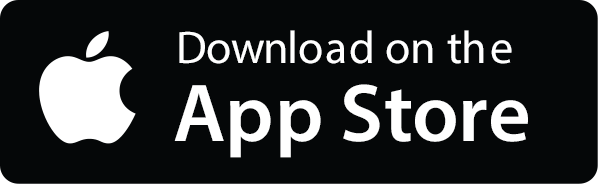
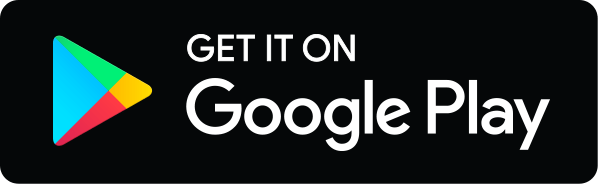