Cardiac Rhythm and Dysrhythmias
Antidysrhythmic drugs are agents that are used to treat disorders of heart rate and rhythm termed dysrhythmias. The normal regular contractions of the heart are initiated and controlled by a specialized conducting system; dysrhythmias occur when the function of this is deranged. To understand the action of the antidysrhythmic drugs it is necessary to understand the actions of this conducting system.
The specialized conducting system in the heart
A heart beat is initiated in the sinoatrial (SA) node by the pacemaker potential. This triggers an impulse, the action potential, which passes across the atrial muscle to the atrioventricular (AV) node and then, via the Purkinje fibres, through the ventricles – coordinating the contraction of the cardiac muscle. The cardiac action potential has a long duration and long refractory period ( Fig. 14.1 ) and differs from one part of the heart to another. The prolonged activation of calcium ion (Ca 2+ ) channels during the action potential plateau in the ventricles maintains Ca 2+ entry, which is essential for the prolonged contraction required for the effective ejection of blood during systole.

The action of the SA node is controlled by autonomic activity.
Pathophysiology
Clinically important dysrhythmias include:
- •
Tachycardia (rapid heart beat)
- –
In the atria (‘supraventricular’ tachycardias): paroxysmal tachycardia, flutter and fibrillation. In atrial fibrillation (the commonest dysrhythmia), the contraction of the muscle is fast, uncoordinated and ineffective; not all impulses get through to the ventricles, resulting in a very irregular heart rate.
- –
In the ventricles: tachycardia, torsades de pointes (a particular type of tachycardia) and fibrillation (the last frequently fatal).
- –
- •
Bradycardia (slow heart beat) and heart block
- –
The latter is due to damage to the AV node or conducting tissue, causing the ventricles to beat at a slower, and often irregular, rate driven by pacemaker activity in the ventricular conducting tissue; an external pacemaker rather than drug therapy is usually required.
- –
Myocardial ischaemia is a common cause of dysrhythmias, which can also be precipitated by reperfusion after an infarction.
The causes of dysrhythmias can be:
- •
Abnormal pacemaker activity : ischaemia-induced depolarization or increased sympathetic activity can cause pacemaker activity to be initiated at an ectopic focus in atria or ventricles.
- •
Early or delayed after-depolarization (EAD, DAD) : these can act as triggers for the generation of abnormal impulses or extra beats.
- –
DADs: the action potential in non-pacemaker cells does not have a phase 4 depolarization (see Fig. 14.1 ), but these cells can become ectopic foci of action potential generation if a phase 4-like after-depolarization occurs. This is associated with increased [Ca 2+ ] i which can be produced by some drugs (cardiac glycosides, norepinephrine).
- –
EADs: these can arise during phases 2 and 3 of the action potential and can themselves trigger propagated action potentials. They occur when repolarization is delayed and the action potential is abnormally long, as occurs during bradycardia. EADs can also be produced by class III antidysrhythmic drugs.
- –
- •
Re-entry : under normal circumstances, the refractory period of the muscle prevents the action potential re-invading the tissue it has just traversed. If, however, there is damage that allows unidirectional propagation, a continuous cycling of the action potential may occur (circus rhythm) ( Fig. 14.2 ). This is more likely in depolarized tissue where Ca 2+ channels rather than Na + channels produce slowly propagated action potentials with a longer refractory period. Re-entry provides an abnormal site for cardiac excitation.
Fig. 14.2
Example of the production of circus rhythm.
A: The normal conduction pattern. B: Unidirectional block and re-entry.
Antidysrhythmic drugs
Actions and mechanisms of action
Most drugs act on the ion channels involved in the various stages of action potential generation and propagation; some act on the sympathetic control of the heart (see Fig. 14.1 and Table 14.1 ).
Example | Mechanism of action | Electrophysiological actions | Clinical use | ||
---|---|---|---|---|---|
Vaughan Williams classification | Class Ia Class Ib | Disopyramide Lidocaine | Na + channel block | Reduced rate of depolarization of action potential, increased ERP, decreased AV conduction | Ventricular fibrillation, especially associated with myocardial infarction |
Class II | Propranolol, atenolol | β-Adrenoceptor antagonism | Slowed pacemaker activity, increased AV refractory period | Dysrhythmia prevention in myocardial infarction; paroxysmal atrial fibrillation due to sympathetic activity | |
Class III | Amiodarone, sotalol | K + channel block | Increased action potential duration and increased ERP | Atrial fibrillation; ventricular fibrillation | |
Class IV | Verapamil | Ca 2+ channel block | Decreased APD, slowed AV conduction | Supraventricular tachycardias; atrial fibrillation | |
Not classified by system | Adenosine | K + channel activation | Slowed pacemaker activity, slowed AV conduction | Given IV for supraventricular tachycardias | |
Digoxin | K + channel activation (vagal action) | Slowed AV conduction (block) | Atrial fibrillation | ||
Magnesium sulfate | ? Ca 2+ channel block | Ventricular fibrillation; digoxin toxicity |
All class I drugs show use-dependence, binding preferentially to sodium ion (Na + ) channels in their activated (open) or inactivated states. The block is thus more pronounced at rates above normal (when the channels spend more time in these states) or in depolarized tissues, where recovery from inactivation is delayed. Class I drugs dissociate at different rates from the channel, resulting in different degrees of use-dependence and leading to subdivision of the class into Ia, Ib and Ic; Ib drugs dissociate rapidly, Ia drugs less rapidly and Ic drugs most slowly. Ic drugs (e.g. flecainide) have been found to cause fatal dysrhythmias and so their use is restricted.
Amiodarone has class III actions and also blocks Na + channels and β-adrenoceptors. These multiple actions may explain its particular efficacy. Sotalol has actions in classes II and III. Adenosine activates adenosine (A 1 ) receptors in the heart to exert the same cardiac effects as acetylcholine interacting with muscarinic receptors. Its short duration of action reduces the likelihood of side effects. Digoxin has direct effects on myocardial contraction due to its inhibition of the sodium/potassium adenosine triphosphatase pump (Na + /K + ATPase), which include increased excitability; this may, in fact, cause the conversion of atrial flutter to fibrillation. However, by increasing vagal action on the heart, it blocks AV conduction and usefully reduces ventricular rate.
Pharmacokinetic aspects
Lidocaine is given intravenously (IV) following myocardial infarction. It is rapidly metabolized by the liver and so is ineffective orally owing to first-pass metabolism; by the same token it has a short half-life (2 h). Propranolol has a half-life of about 4 h with significant first-pass metabolism: its antidysrhythmic activity is maintained by an active metabolite. Amiodarone is extensively bound in tissues and only slowly eliminated; its half-life is 10–100 days. Verapamil is orally active and has a half-life of 6–8 h. Adenosine is given IV to terminate supraventricular tachycardia and acts for only 20–30 s due to rapid uptake into erythrocytes and subsequent metabolism.
Unwanted effects
Antidysrhythmics generally have a narrow therapeutic index. As most antidysrhythmics are metabolized by the cytochrome P450 system, pharmacokinetic interactions involving enzyme induction or inhibition may be clinically significant. Ca 2+ channel blockers should be avoided in heart failure because of reduction in contractility. Class II drugs should not be used in subjects with asthma as they can precipitate bronchoconstriction. Amiodarone has important adverse effects, not only triggering torsades de pointes (shared with other class III drugs), but also exhibiting thyroid and pulmonary toxicity.
Vernakalant is a recently introduced anti-arrhythmic drug which is used IV to treat atrial fibrillation. It acts by delaying atrial repolarization by blocking atrial selective potassium and sodium channels.
Blood Pressure Control and Hypertension
The cardiovascular system is regulated by complex mechanisms, the main variable under control being the arterial blood pressure, which is the driving force for the flow of blood through the systemic circulation. Fig. 14.3 gives a rough outline of the principal homeostatic factors controlling the arterial pressure. The arterioles play the major role in determining the peripheral resistance and also regulate the relative blood flow through individual organs. The cardiac output depends on the stroke volume (SV) and the heart rate . The main factors affecting the SV are the plasma volume and the venous return ; the heart rate is controlled primarily by the sympathetic nerves (which increase the rate) and the parasympathetic nerves (which decrease the rate). Note that the kidney plays a vital part in blood pressure control as do some paracrine factors including nitric oxide and prostacyclin (PGI 2 ) released by vascular endothelial cells (which increases cyclic guanosine monophosphate, cGMP), adenosine and prostaglandins and bradykinin generated during inflammatory responses.

The boxes give the main antihypertensive drugs.
Vasodilatation involves an increase in cyclic adenosine monophosphate (cAMP) in vascular smooth muscle and is brought about partly by adrenaline acting on β 2 -adrenoceptors. (A decrease in sympathetic tone can also produce vasodilatation.)
Vasoconstriction involves an increase in intracellular Ca 2+ and is brought about primarily by noradrenaline (NA) released from sympathetic nerves acting on α 1 -adrenoceptors (for more detail, see Chapter 11 ). Vasoconstriction can also be regulated by angiotensin II and local release of endothelin from endothelial cells.
Pathophysiology of hypertension
The main pathological condition affecting the vascular system is hypertension (high blood pressure). This can be caused by renal disease, endocrine disorders or phaeochromocytoma (a catecholamine-secreting tumour; rare), but in most patients the cause is unknown and the hypertension is termed ‘essential’ (for archaic and mistaken reasons). From Fig. 14.3 , it is clear that a multitude of factors influence arterial blood pressure, all of which could be implicated in the pathogenesis of hypertension, although this is mainly caused by the kidney and/or the sympathetic nervous system. If hypertension is not treated, coronary thrombosis, stroke or kidney failure may result.
Antihypertensive drugs
The main drugs are:
- •
agents affecting the renin–angiotensin system (angiotensin-converting enzyme (ACE) inhibitors, angiotensin (AT) I receptor antagonists, see below);
- •
the thiazide diuretics ( Chapter 18 );
- •
calcium antagonists (see below);
- •
β-Adrenoceptor antagonists (see below and Chapter 11 ).
In young patients, initial treatment is with agents affecting the renin–angiotensin system; older patients are started on thiazides or a calcium antagonist.
Other drugs that can be used include:
- •
drugs acting centrally or peripherally on noradrenergic transmission: doxazosin, clonidine, methyldopa; these are dealt with in Chapter 11 , Chapter 12 ;
- •
vasodilators (covered briefly below).
Thiazide diuretics
Examples: bendroflumethiazide, hydrochlorothiazide.
Actions relevant to hypertension:
- •
Increase in salt and water excretion and thus reduction of extracellular fluid volume.
- •
Decrease in cardiac output through reduced plasma volume.
- •
Reduction in peripheral resistance (an as yet unexplained effect).
- •
Increase in renin release (which may counteract some of the above effects on the blood pressure).
Thiazides have antihypertensive actions when used alone and also potentiate the action of other antihypertensive drugs.
Drugs acting on the renin–angiotensin system
The sequence of events is outlined in Fig. 14.3 . (AT III, a breakdown product of AT II, also stimulates aldosterone secretion; not shown.)
The release of renin, a proteolytic enzyme, is stimulated by:
- •
decreased blood flow through the kidney,
- •
reduced Na + concentration in the distal tubule,
- •
β-adrenoceptor agonists.
The renin–angiotensin system tends to increase blood pressure by:
- •
the vasoconstrictor action of AT II (partly through augmentation of NA release);
- •
the aldosterone-mediated retention of salt and water, which leads to increased extracellular fluid volume. The raised plasma volume increases cardiac output.
These effects can be decreased by ACE inhibitors and by AT 1 receptor antagonists, the sartans. In addition to their action as antihypertensives, these two groups of drugs are also used in heart failure and diabetic nephropathy.
ACE inhibitors
Examples include: captopril, lisinopril and enalapril.
Actions relevant to hypertension
ACE inhibitors cause a fall in blood pressure affecting particularly the vasculature of the brain, kidney and heart. The drop is more marked in hypertensive individuals, especially if diuretics are also being given. They are given orally, lisinopril once a day and captopril twice a day and are excreted in the urine.
Main unwanted effects
- •
A dry cough.
- •
Hypotension (initially; particularly in the presence of diuretics).
- •
Recent work suggests that chronic use of this drug class may have an increased risk of lung cancer.
Contraindications
- •
Renovascular disease.
- •
Pregnancy.
Sartans
Actions relevant to hypertension
These drugs antagonize the action of AT II on the AT 1 receptors thus decreasing the effects of AT II (see above).
Calcium antagonists
Examples are: nifedipine, amlodipine.
The calcium antagonists block Ca 2+ entry through Ca 2+ channels. Action relevant to hypertension is the inhibition of depolarization-induced Ca 2+ entry into cardiac and vascular smooth muscle. The vasodilator effect reduces arterial pressure. They cause flushing and ankle oedema; the absence of more serious unwanted actions is a recommendation for their use as antihypertensives.
β 1 -Adrenoceptor antagonists (sometimes referred to as cardioselective)
Examples: atenolol, metoprolol.
Actions relevant to hypertension
- •
Decrease in cardiac output.
- •
Decrease in sympathetic activity by an action in the CNS.
- •
Decrease of renin release, which reduces the generation of AT I and II and thus decreases AT II-induced vasoconstrictor activity and aldosterone-induced salt and water reabsorption.
Vasodilators
Various vasodilator agents have been used as antihypertensives (and are sometimes still so used in special circumstances).
Minoxidil is a K + channel activator which relaxes vascular smooth muscle by hyperpolarizing the plasma membrane, thus preventing Ca 2+ entry through voltage-dependent Ca 2+ channels. It is used with a β-adrenoceptor antagonist and a diuretic for very severe hypertension.
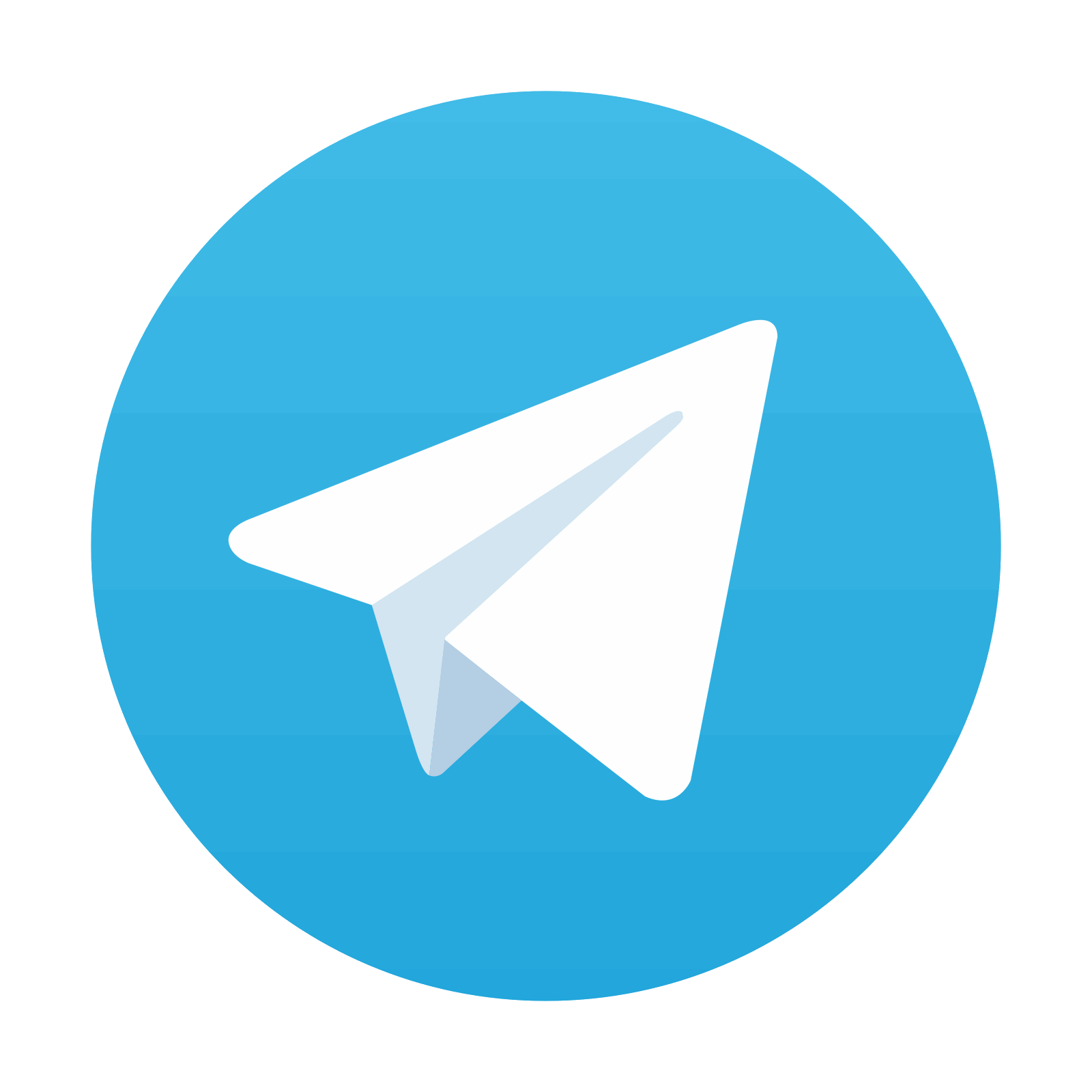
Stay updated, free articles. Join our Telegram channel

Full access? Get Clinical Tree
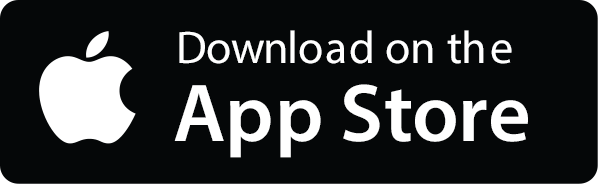
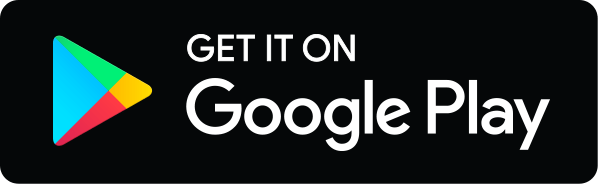