Detonation of a Nuclear Device
Cham E. Dallas
INTRODUCTION
The increasing likelihood of the use of weapons of mass destruction (WMD) on large civilian populations has been described in international government alerts (1), U.S. congressional hearings (2) and research studies (3), and numerous scientific publications. The Islamic terrorist attacks on New York and Washington, D.C., have accentuated the reality of this threat. There is continued concern over the security of the enormous arsenal of nuclear, chemical, and biological agents left over in Russia as a result of the Cold War. It is known that Libya, Iran, Syria, Iraq, and North Korea have been actively recruiting the scientists who constructed this massive stockpile, and it is not certain where many of these experts are now (4). In just the case of biological weapons, at least 17 countries are believed to be in possession of or actively developing these agents (5). Although the terrorist use of nuclear weapons has a certainty of mass casualties, empirical analysis of the historical trends of terrorist events have shown that chemical and biological agents were employed far in excess of nuclear or radiological materials (6).
As the threat of the use of WMD on the civilian population increases, data suggest that hospitals and medical providers in general are significantly unprepared for a biological, chemical, radiological, or nuclear incident, with a nuclear weapon attack presenting the greatest degree of a lack of preparedness. Radiological attacks are defined as involving the release of radioactive materials into the proximity of people without a nuclear explosion and typically involve far fewer and less consequential casualties than nuclear detonations. Only in some regions of the former Soviet Union have there been significant casualties from the release of radionuclides into the environment without atomic detonation (7,8). Use of a nuclear weapon will involve a nuclear detonation, with the accompanying massive explosion, devastating fireball, extensive burns in patients, trauma victims, blindness, and short- and long-term radiation sickness. This chapter focuses on specific medical response procedures for treating patients resulting from the use of nuclear weapons, including the unique aspects of dealing with mass casualties.
Although the preparation of hospitals using the principles inherent in hazardous materials (hazmat) training has provided some degree of response capability, there are significant additional concepts for an overwhelming impact on public services. Medical as well as law enforcement personnel will need to understand the unique challenges in dealing with the intense public fear of radiation, which will significantly impact on the apprehension of perpetrators as well as maintaining public order (especially in nuclear attacks, with the attendant widespread destruction). Public health officials will learn of the potential radiological monitoring of patients and the environment, dealing with the likelihood of a large number of “worried well,” transportation difficulties inherent in mass casualty management, and the sheer magnitude of nuclear attacks in general. Pharmacists must understand their critical role in the rapid dispersion of iodide tablets for the prevention of radiation-induced thyroid cancer, the pharmaceutical agents of most importance in nuclear attack medical response, and the agents for removal of internal radioactive contaminants from exposed patients.
THEORETICAL CONCERNS RELATED TO NUCLEAR BLAST
Radiation exposure involves various particles (i.e., beta, alpha, neutrons) or rays (gamma) generated from the unstable elements released into the environment (see Chapter 13). Gamma rays and some of the more intense beta particles (electrons emitted from radioactive materials) can be detected at a distance, and scanning the individuals and samples taken from them can generate relatively rapid indications of exposure. Unfortunately, alpha particles (a low-penetration particle consisting of two protons and two neutrons) cannot be detected with standard detection devices, so plutonium exposures, for example, are harder to detect. Because nuclear weapons generate a wide range of radiations and radioactive particles, the scanning of patients and the environment for gamma and beta detection is sufficient to determine an overview of the exposure potential for an individual patient.
TABLE 18-1 Patient Categories Based on USSR Chernobyl Classification | ||||||||||||||||||||||||||||||||||||||||||||||||||
---|---|---|---|---|---|---|---|---|---|---|---|---|---|---|---|---|---|---|---|---|---|---|---|---|---|---|---|---|---|---|---|---|---|---|---|---|---|---|---|---|---|---|---|---|---|---|---|---|---|---|
|
Classification of both nuclear detonation and radiological contamination patients in the event of a nuclear detonation is significantly expedited by evaluation of hemodynamic parameters, as well as the time of prodromal onset, as discussed in Chapter 16 (10). The rapid decline in blood lymphocytes induced by significant radiation exposure is particularly useful. Data gathered for the large number of highly exposed cleanup workers (known as “liquidators”) and others following the Chernobyl nuclear disaster provide an example of the classification of radiation patients as to outcome (Table 18-1). Lymphocyte counts were found to be highly correlated with the treatment categories, with very low numbers found in patients who did not survive and progressively higher counts found in groups that had probable survival with treatment, possible survival without treatment, and probable survival without treatment, respectively. Granulocytes had a similar utility and over a larger range of values (11). Platelets were useful in distinguishing between the lower exposed groups but had less utility in distinguishing between higher exposed groups (12). If hemodynamic parameters are not available or of questionable quality, the onset of the prodromal syndrome will still have considerable utility in patient classification. Indeed, prodromal onset may be better correlated with radiation dose than blood parameters. If both are available, they can be used to verify each other in patient category designation. The health provider will have to be careful in using vomiting as a parameter, however, because it is likely that many patients will present this symptom for psychological reasons (13). Generally, patients with very low or undetectable lymphocyte counts, prodromal onset of less than 30 minutes, and very severe (i.e., 60% of the body) burns are likely to be in the expectant category. Every case should be evaluated separately, however, on the judgment of the provider on site (Table 18-1).
TABLE 18-2 Radiation-induced Cancer Induction Following Atomic Bomb and Nuclear Reactor Airborne Releases | ||||||||||||||||||||||||
---|---|---|---|---|---|---|---|---|---|---|---|---|---|---|---|---|---|---|---|---|---|---|---|---|
|
One of the most feared long-term effects of radiation exposure is the subsequent induction of cancer (14,15). It has been found after tracking survivors of the Hiroshima and Nagasaki atomic bomb attacks that there is a series of long latent periods followed by a detectable incidence of radiation-induced cancers (Table 18-2). Because some of the nuclear weapons available today have far greater yields than the atomic bombs used on Hiroshima and Nagasaki, the degree of cancer incidence may be considerably greater. Even the latent periods may be shorter, as was seen following the Chernobyl nuclear accident with the much shorter incidence (presumably due to the much higher dose rates for radioiodine) for thyroid cancer (16,17) (Table 18-2).
UNSUBSTANTIATED FEAR OF RADIATION-INDUCED BIRTH DEFECTS
One of the most entrenched concepts in the general public concerning radiation is the fear of birth defects induced by radiation exposure. Certainly, it has been shown that intense x-ray exposure has produced birth defects in humans (17,18,20) and there have been a very limited number of defects reported in Hiroshima and Nagasaki atomic bomb survivors (21,22,23). Indeed, many of these survivors were avoided socially in relation to marriage selection because of a fear of birth defects in subsequent offspring. However, the actual incidence of teratogenicity resulting from radiation exposure has been highly overrated, based on numerous historical radiation exposures worldwide (24).
The most distinctive example of how this fear of birth defects impacts on a medical response to ionizing radiation was the Chernobyl experience (25,26). Over 100 times the amount of radioactivity generated by both the Hiroshima and Nagasaki atomic bomb detonations was released into the air after the Chernobyl nuclear accident (27). In the immediate aftermath of the accident, over 30,000 women terminated their pregnancies directly as a result of fear of the birth defects from their radiation exposure. These are women who would have had the babies otherwise, so their decision (supported by medical providers) was completely due to the accident. Subsequent analysis of the 60,000 other equally exposed women who did have their babies, however, revealed no statistically significant incidence of birth defects. Clearly, the information given to the women concerning relative risk of birth defects was false and misleading. In conjunction with the very low incidence of birth defects after the atomic bomb detonations in Japan, a fear of significant numbers of birth defects is not justified. In both the immediate aftermath and long-term medical response to nuclear weapon use and in radiological exposures, therefore, termination of pregnancy due to the anticipation of radiation-induced birth defects is not a scientifically justified course.
DETECTION AND MEDICAL SURVEY FOLLOWING NUCLEAR BLAST
Radiation detection was discussed in Chapter 14. Radiation exposure to humans can result from irradiation from a distance, contamination on the skin, hair, and clothing, and from internal contamination of radioactive materials from inhalation or ingestion (28). In a long-distance irradiation (gamma, beta, and neutron), internal injury can occur due to the penetrating power of the particular radiation. One intense source of this external irradiation occurs in the blast of radiation emitted from a nuclear weapon at the time of detonation, and decreases in intensity (not counting shielding) are evident with distance from ground zero. Evaluating an individual for possible contamination or radiation emission using detection devices is referred to as a radiation survey. A radiation survey does not, except for some neutron-induced activity, detect a person’s exposure to radiation. A radiation survey is useful for the radiation that occurs as a result of fallout or the contamination from radiological exposure. Therefore, the radiation survey helps identify those individuals who have been exposed and is invaluable in protecting those serving the victims (and preventing further exposure). It can only be expected, though, to give an approximation of the extent of exposure that actually occurred (29).
If the emergency unit does not possess a gamma-counting device, state and federal authorities must be contacted and a detection instrument procured immediately. Along with survey instrumentation, at least one trained person should accompany the device, and this training must be transmitted to as many personnel on site as quickly as possible upon arrival. Fortunately, basic radiation survey can be conveyed to a wide variety of personnel fairly quickly, although the overall process must be under the authority of the incident commander and the local physicians and other health care professionals. If patients have detectable radiological contamination, their clothes must be removed, and these individuals examined and treated as potential radiation victims.
In a radiological terrorism event, as well as in some accidental radiation exposures, the specific radionuclide used determines the specificity and efficacy of scanning and detection (30). If strontium-90 was the agent of concern, for example, the radiation monitoring would have to focus on beta detection. From the consideration of the penetration potential of various radiations, gamma and neutron radiation have the highest penetrating power (i.e., through walls), beta is less penetrating (i.e., most will not pass all the way through the body), and alpha particles will not even penetrate a piece of paper (29,31). However, penetrating power only determines the mode in which the various radiations can exert physiological injury. Gamma and beta radiation can be a health hazard from a distance due to their penetrating power. Although alpha particles would not be dangerous outside the body (i.e., on clothing), once they are inhaled or ingested into the body, they have a very high radiation energy with a significant potential of generating toxic injury in the tissues immediately next to them.
The initial radiation survey technique is provided in Table 18-3 and describes a generalized overview of the approach to be taken in surveying patient populations for radiation exposure and potential decontamination. Whenever possible, a health physics practitioner should be included in the radiation survey team. Because properly trained individuals are few in number, this may be hard to accomplish if there is a large-scale event. The extent of the survey would depend to some extent on the number of potential victims as well as the size of the medical response team. Whenever time and resources are sufficient, and especially when the patient population is lower, the survey should be conducted as thoroughly as possible. This radiation survey is not specific only to nuclear detonation but may be used in any radiation exposure (Table 18-3).
Monitoring of clothing is usually considered to be extraneous to the mission, and the clothing should be
bagged for later disposal. It is important to protect the probe from contamination with a glove because in most emergency settings a replacement will not be available. Therefore, complete protection of the probe becomes a serious limiting factor. If the monitoring crew has sufficient confidence, and the patient is very cooperative, one might consider using the probe without the glove. This would increase the sensitivity of the probe for weak beta particles and perhaps some alpha contamination. However, the most important consideration is to protect the probe for subsequent use (which in mass casualty situations would mandate the use of a glove even with the availability of multiple probes). Checking the background of the examining area should be done at periodic intervals in order to remove the confounding interference with the patient measurements. For every patient, a record of his or her contamination (or lack thereof) should be made for future reference. A simple schematic of body surfaces (front: with top of feet shown; back: with bottom of feet shown; right face; and left face) would be used to indicate the presence (and absence) of contamination. The type of survey meter shown and its settings should also be indicated, along with the patient name, date, and time.
bagged for later disposal. It is important to protect the probe from contamination with a glove because in most emergency settings a replacement will not be available. Therefore, complete protection of the probe becomes a serious limiting factor. If the monitoring crew has sufficient confidence, and the patient is very cooperative, one might consider using the probe without the glove. This would increase the sensitivity of the probe for weak beta particles and perhaps some alpha contamination. However, the most important consideration is to protect the probe for subsequent use (which in mass casualty situations would mandate the use of a glove even with the availability of multiple probes). Checking the background of the examining area should be done at periodic intervals in order to remove the confounding interference with the patient measurements. For every patient, a record of his or her contamination (or lack thereof) should be made for future reference. A simple schematic of body surfaces (front: with top of feet shown; back: with bottom of feet shown; right face; and left face) would be used to indicate the presence (and absence) of contamination. The type of survey meter shown and its settings should also be indicated, along with the patient name, date, and time.
TABLE 18-3 Basic Radiation Survey Technique for Patients | |
---|---|
|
Further radiological surveys are usually beyond the capabilities of most emergency settings, but additional information gathered can be useful to subsequent treatment. Blood samples can be taken and lymphocyte counts made over time, which would be plotted out on an Andrews nomogram (Chapter 16). This profile would provide an indication of the likely impact on the blood elements of the affected individual after the initial crisis is over. Whole-body counting can be employed to determine the extent of internal radionuclide contamination in patients, after decontamination. These counters are relatively scarce but have considerable utility in delineating patient dosimetry for long-term health care planning. Additional scarce, but highly useful techniques can also be employed in long-term follow-up, such as lymphocyte cytogenetics, fixed in situ hybridization (FISH) techniques, “chromosome painting,” and electron spin resonance. Chromosome analysis for dicentric malformations was described in Chapter 16. Expertise and equipment for these approaches can be identified by contacting the Armed Forces Radiobiology Research Institute (AFRRI) in Washington, D.C., or the Lawrence Livermore Laboratory in San Francisco.
NUCLEAR WEAPON DETONATION
With the demise of the Cold War, the possibility of devastating attacks with multiple nuclear weapons (known as mutual assured destruction, or MAD) has decreased dramatically. However, the new asymmetric threat for a nuclear weapon involves small terrorist groups or rogue states detonating a single nuclear weapon in a population center using covert delivery (e.g., a delivery van). The “nuclear club” of countries possessing nuclear weapons now includes North Korea, Pakistan, possibly Iran, and other nation-states who have been willing to export this technology freely. Although there has been a precipitous decline recently in the number of active nuclear warheads in the U.S. and Russian nuclear arsenals, it is unfortunate that none of the weapons-grade material removed from the deactivated warheads has been destroyed. Instead, the plutonium and uranium pits from these thousands of nuclear warheads have simply been stored away. Possible theft of small portions of the tons of this stored nuclear material, especially from the Russian arsenal, is a constant source of concern. Additional efforts by unstable and even aggressive nations (e.g., North Korea, Iran) to produce nuclear material for use in warheads also heightens the tension.
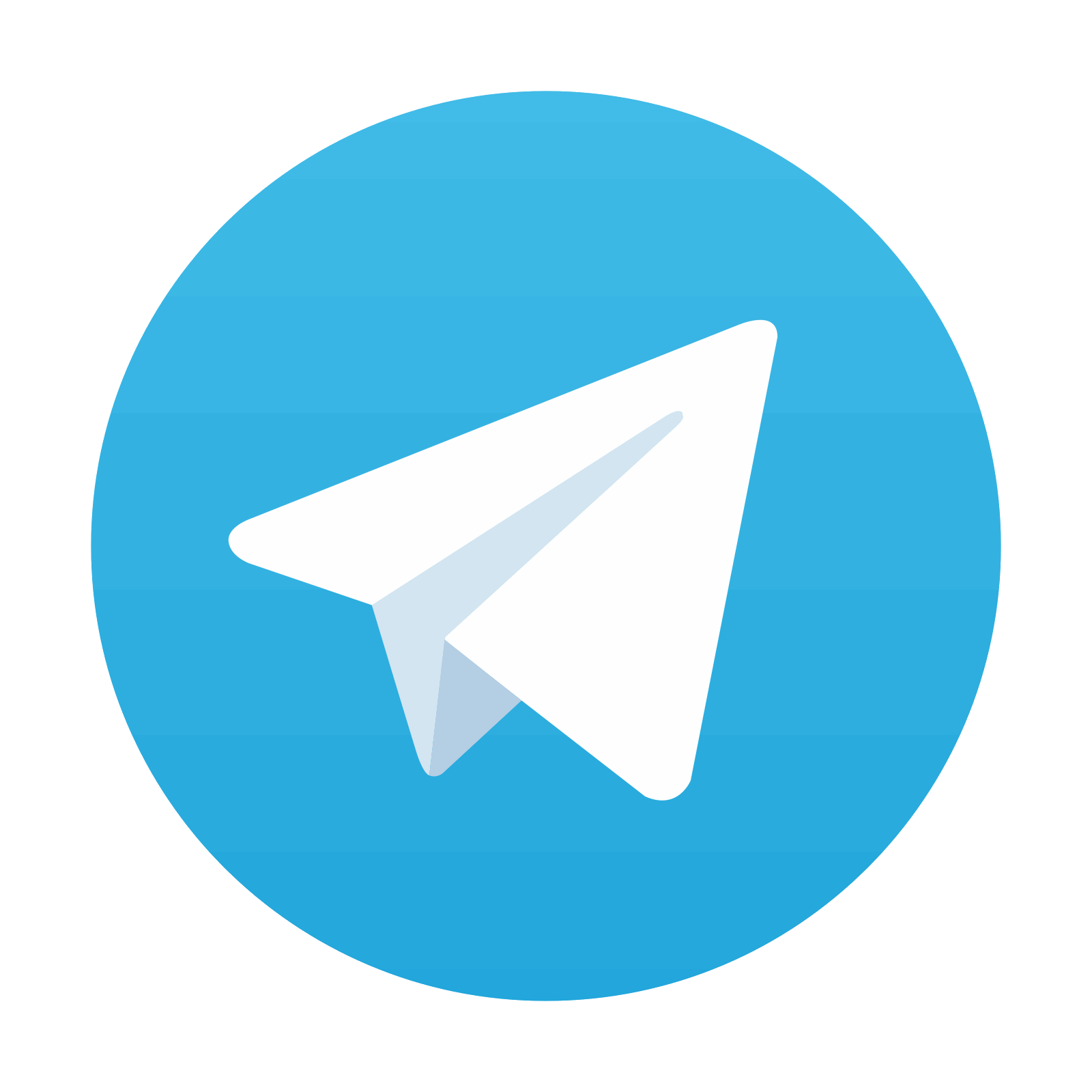
Stay updated, free articles. Join our Telegram channel

Full access? Get Clinical Tree
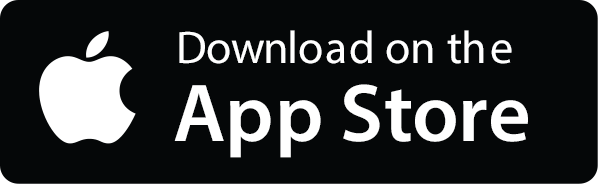
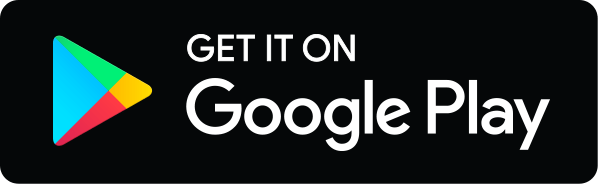