Detection, Prediction, and Surveillance
Warren L. Whitlock
INTRODUCTION
Surveillance is an all-encompassing activity that includes planned and unplanned information collection, statistical analysis, and the prediction reporting of possible future events. This definition encompasses the next generation of detection and surveillance systems on the horizon and provides the groundwork for both horizontal and vertical views of the requirements for successful surveillance systems. The Centers for Disease Control and Prevention (CDC) defines public health surveillance as “the ongoing, systematic collection, analysis, and interpretation of data (e.g., regarding agent/hazard, risk factor, exposure, health event) essential to the planning, implementation, and evaluation of public health practice, closely integrated with the timely dissemination of these data to those responsible for prevention and control” (1). Prediction of health trends depends on accurate detection data, dynamic data integration for surveillance, and Bayesian probability for sensitivity, specificity, and statistical accuracy. Identifying the requirements, components, and systems engineering for early detection of health states can provide a strategic decision capability concerning resources and disease prevention actions. However, surveillance systems can have severe shortcomings, based on the quality of the data collected and the detection methods used to provide surveillance. These are essential topics for a discussion of detection and surveillance capabilities and realities.
Although the CDC provides a reasonably clear general definition for health surveillance, it does not provide a specific definition of “detection,” and the key to successful surveillance is early detection. Surveillance of specific detection information, when recognized, allows for early strategic intervention. Detection methodology is the historical foundation of epidemiology and surveillance and the primary key for effective actions to limit, control, and prevent potential disease outbreaks. Surveillance can be either accurate and valuable or inaccurate with little utility, based on its precision and accuracy for prediction of “true positive” public health outcomes.
One of the finest examples of detection and surveillance is Dr. John Snow’s epidemiological research in 1854 in detecting cases of cholera and their location in London. Each cholera case detected and its location were painstakingly plotted by hand on a map of the city. The density of cholera cases in a concentric fashion based on their location made the association and conclusion obvious to anyone that it was the centrally located town well and its water that was contaminated with cholera. The mapping of each case detected provided for a surveillance system to understand the relationship between the disease and the source to form strategic decisions regarding resources and public health policy, which later formed the basis for modern epidemiology and public health activities. Snow’s work constituted a case-controlled study demonstrating that people from low-risk areas outside of London who drank the cholera-contaminated water developed cholera. This confirmed his theory that cholera cases were associated with the well water before medical science had discovered it was a bacterial organism that caused the contamination and disease (2). It was the undeniable confirmation of Snow’s surveillance prediction that caused a revolution in public health attitudes. Despite the profound effect of this methodology, it took almost 20 years to fully change the public behavior even after the causes and effects of cholera were known.
History teaches us that the ability to predict future health events from detection and effectively communicate this information to leaders can be devastating if not rooted in the daily management and administration of our government system. The Native Americans taught the early British and European settlers much important information, but perhaps no lesson from that period of history is as poignant as what was learned from the first recorded incidence of biological warfare use in North America. The French and Indian War was waged from 1754 to 1763. After seven years, a victory was still not foreseeable until a British commander approved a submitted plan to use blankets from a smallpox hospital to infect Native American tribes that had no natural resistance. William Trent, a commander of the local militia of the townspeople of Pittsburgh, notes in his journal on May 24, 1763, “[W]e gave them two Blankets and an Handkerchief out of the Small Pox Hospital. I hope it will have the desired effect” (3).
Without detection and surveillance capability, smallpox spread rapidly across the Native American tribes. After the initial cases, the true cause went unrecognized by Native
American leaders, and the morbidity and mortality resulted in devastation of the eastern Native American population, effectively ending the French and Indian War in less than a year. Native Americans continued to suffer from smallpox outbreaks for years afterward because they were not capable of understanding the public health strategic consequences.
American leaders, and the morbidity and mortality resulted in devastation of the eastern Native American population, effectively ending the French and Indian War in less than a year. Native Americans continued to suffer from smallpox outbreaks for years afterward because they were not capable of understanding the public health strategic consequences.
Detection and surveillance requirements for protection of public health against weapons of mass destruction (WMD) will be no less an important requirement than the understanding of the contagion risk of smallpox might have been to Native Americans. The changes required in the United States to achieve effective detection and surveillance will be no less profound than what Snow faced in changing public health policy after discovering the relationship between cholera and water contamination. The understanding and transformational change in a global health detection and surveillance system required to prevent or limit the impact of a biological or chemical terrorism attack effectively could result in even more lives saved today than all the lives saved in history because of Snow’s original discovery concerning cholera.
PREPAREDNESS ESSENTIALS
The possibility of the intentional spread of chemical, biological, radiological, nuclear, or explosive (CBRNE) agents by terrorists makes it essential that preparedness planning incorporate the use of advanced technology for medical surveillance. This must be done in support of a wide spectrum of professions to include first responders, public health officials, and military services (both active and reserve components) and by commercial companies that import products into the United States. Detection and surveillance for threat and risk reduction needs specifically to target CBRNE and, most recently, “cyber-terrorism attacks” (4). If surveillance is to be effective, it must be established within the federal standards for medical data but support the systematic processes of data collection, analysis, and dissemination among local, state, and federal agencies.
“All data concerning global disease incidence, including WHO data, should be treated as broadly indicative of trends rather than accurate measures of disease prevalence” (5). To further define the critical requirements for detection and surveillance, the real challenge is to distinguish among a disease outbreak, the deliberate use of biological weapons, andthe native fluctuations in the detection system that are not predictive of either one. Early detection presupposes there is a system matrix for surveillance that can then predict future health events. Without the ability to discriminate between these incidents at an early point in time through detection, surveillance will only occur in a retrospective, asynchronous, postmortem fashion and result in “lessons learned” as a history archive footnote. Efforts under way on both the civilian and military community homeland security fronts and defense and preparations for potential bioterrorism agent deployment will have clear and measurable benefits to our current health surveillance systems used by the Department of Defense (DoD) and the CDC today.
In October 2000, the Defense Threat Reduction Agency’s Advanced Systems and Concepts Office (ASCO) and the CDC cosponsored a workshop aimed at identifying the essential elements of a national health surveillance system. The National Health Surveillance Workshop described three types of surveillance systems: “data mining, rapid diagnostics, and syndromic systems” (6).
The data mining process is performed retrospectively and uses statistical methods to uncover relationships that otherwise would not be discovered or understood. Data mining evaluates existing data that is already archived, and it requires no effort to perform collection activities. But because it is retrospective, it is limited even in the most ideal of circumstances to providing information on “what happened in the past.” Data mining cannot by itself provide the necessary prediction of trends needed for surveillance systems. The analogy would be to drive a car forward by only having the ability to look backward to see where you have been. Data mining also depends on the quality of legacy data recorded, which for most available surveillance systems is based in medical billing codes (ICD-9). In medical systems, this is the most prevalent methodology used for surveillance today. Other “legacy” data models are being explored, such as emergency department “chief complaint” or patient call-in information lines, but because of inconsistent definitions of the data and lack of systematic collection, they are all limited in meeting true surveillance requirements.
Because many of the CDC’s identified high-risk bioagents have zoonotic or animal origins, surveillance systems must use more than just human insurance billing codes and incorporate standards in broad categories of agriculture, animal, and human data for aggregation. Future surveillance systems must include veterinary medicine, agricultural system data, and U.S. Trade and Immigration information, as well as medical health data.
Just as the CDC has classified bioterrorism agents/diseases by category (7), the International Office for Epizootics (OIE) has classified animal diseases into categories based on their risk of negatively impacting international trade and global economy. The class A list is defined by “Transmissible diseases which have the potential for very serious and rapid spread, irrespective of national borders, which are of serious socio-economic or public health consequence and which are of major importance in the international trade of animals and animal products.” The class B list is defined by “Transmissible diseases, which are considered to be of socio-economic, and/or public health importance within countries and which are significant in the international trade of animals and animal products” (8). Bioterrorism agents such as anthrax, Q fever, tularemia, brucellosis, and glanders are on both the CDC and the OIE lists.
The spectrum of detection systems to support surveillance must include environmental, agricultural, and occupational field detection evidenced by the growing number of stationary and mobile rapid detection and diagnostic devices (RD3) that provide immediate identification of biological, chemical, and radiation agents. RD3 can provide real-time detection with a high degree of sensitivity in multiple sites over long periods of time. However, the RD3s still need an integrating system for surveillance prediction of events using multiple disparate data sources that yield a high probability and accuracy. These types of systems are generally more expensive and more sensitive to environmental conditions and therefore of limited utility because they are subject
to variable specificity in the detection thresholds. The validation of alarm thresholds and higher quality devices for proven field use is needed before deploying these systems as part of surveillance systems in real-world operations.
to variable specificity in the detection thresholds. The validation of alarm thresholds and higher quality devices for proven field use is needed before deploying these systems as part of surveillance systems in real-world operations.
The importance of research and technology enhancement of medical surveillance is clear from the largest retrospective investigation injury and disability studies in DoD. This work took 9 years to complete by review of medical records and resulted in these five recommended steps for surveillance system development:
Surveillance: Determine problem to be investigated.
Research: Identify the specific data for cause and risk factor detection.
Research/intervention trials: Use for prevention and surveillance strategies.
Policy, behavioral changes, and equipment: Optimize the use of technology for implementation.
Ongoing surveillance and research: Evaluate effectiveness of future planning and forecasting capabilities (9).
The requirement for “Research” is listed in three of the five categories from this study compiled by military and civilian experts. The most notable conclusion concerning detection and surveillance is that current systems are not adequate to meet our national, state, or local agency requirements for early detection, accurate prediction, and valid decisions concerning resources by public health, federal, and state government officials.
The two categories of clinical surveillance are direct and indirect methodology. Indirect surveillance is analogous to taking a census based on current information systems and medical codes. This can provide general information concerning past or current events but by itself it cannot necessarily provide for early or accurate identification of future trends. The direct type of surveillance is more formal because of its specific data collection and examination to determine factors or conditions that predict health events in a given population, location, and time period. An example of indirect surveillance is when the recorded atmosphere and weather conditions monitored from satellite by NASA were combined with recorded outbreaks of the West Nile virus in Egypt. Matching of these historical trends showed an association of flooding of the Nile River during the summer season to epidemics of West Nile virus infection. Because mosquitoes are the carrier and vector of the West Nile virus, pooled water and warm seasons provide conditions necessary for West Nile epidemics. Public health actions to address these conditions were based on the understanding of this indirect surveillance and subsequently were proven to reduce the number of cases of West Nile virus infection (10). This type of surveillance of environmental conditions provided valid prediction of human and animal disease risk in large geographical areas and populations, which are used now in other areas of the world with West Nile virus infections. The ability to collect clinical information within our indirect medical information system must have the capability to use patient symptoms and signs, or what is known as “syndromic surveillance.”
Serological surveillance in populations is a more specific measure, which can be conducted under both direct and indirect methods. Indirect surveillance is similar to the monitoring of HIV-positive rates routinely reported to public health and the CDC. Direct surveillance is illustrated by the specific and systematic monitoring for coronavirus (cause of the severe acute respiratory syndrome [SARS] epidemic), the seroconversion rate in both human and animal populations to predict background population exposure, predict risk for potential epidemic, and institute tighter infection control policies in high-risk countries (11). Because one of the critical predictors of epidemics is a population’s native resistance to a disease, serological data can also have great value for epidemic probability and forecasting. Direct surveillance of serological conversion rates in a targeted population can provide evidence of subclinical disease exposure, risk of disease epidemic, and assist with prediction information for other areas and populations. If direct surveillance for serological conversion is used in a large target population, it can have very high costs and present implementation problems for training and education across many organizations. Both direct and indirect serological surveillance methods can provide predictions of prevalence and risk in geographical distributions for many human and veterinary diseases. Serological surveillance provides objective information concerning the frequency of specific findings in a population and is therefore more quantifiable than subjective billing code surveillance. For medical surveillance to be used effectively by health care providers and decision makers, it must be available in a near “real-time” capability for accurate probability prediction. This requirement provides an opportunity for technology and equipment to support a “next-generation” surveillance capability to deter and prevent potential terrorist attacks and deployment of bioterrorism agents. This chapter provides a glimpse of some near-term and long-term products that are in development for both civilians and military.
PREPAREDNESS HISTORIC EVENTS AND CASE HISTORIES
The concept of a modern biological warfare and research development program was developed after World War II when investigations showed evidence that Japan had conducted systematic and comprehensive research on potential biological agents for use during the war. Records show the testing of these agents on human prisoners, the modifying and weaponizing of the bioagents, and the development of delivery systems, such as aerosol delivery of anthrax spores (12). The U.S. research efforts on the atomic bomb ended the war with Japan before these biological research efforts could come to fruition and be used against the Allied forces.
The dropping of the atomic bomb at Hiroshima and Nagasaki ended not only the war but the deployment of Japan’s considerable biological warfare capability. Planning the ideal future surveillance system might be based on determining the requirements to meet the worst case scenario.
Therefore the “ideal surveillance system” would provide enough sensitivity and specificity for early detection, accurate prediction, and effective preventive actions in preparedness against the “ideal CBRNE agent.” The ideal syndromic surveillance system should be simple to use, supplied at no
or low cost to end users, and be of great value in their job performance, but still provide for data collection at point of care in a wide range of settings (public health clinics, physicians’ offices, emergency departments, pharmacies). It would also provide for alert thresholds and transmission (data synchronization) of information and alerts across jurisdictions, regions and states, and network domains. This type of system would be able to provide data query over time for valid predictions of trends that lie outside of historical trends and provide indications for in-depth ad hoc query and investigation.
or low cost to end users, and be of great value in their job performance, but still provide for data collection at point of care in a wide range of settings (public health clinics, physicians’ offices, emergency departments, pharmacies). It would also provide for alert thresholds and transmission (data synchronization) of information and alerts across jurisdictions, regions and states, and network domains. This type of system would be able to provide data query over time for valid predictions of trends that lie outside of historical trends and provide indications for in-depth ad hoc query and investigation.
Asynchronous data is analyzed retrospectively, whereas synchronous data enable the concept of real-time information analysis. Microsoft defines real-time data (RTD) as data that updates on its own schedule (for example, stock quotes, manufacturing statistics, Web server loads, and warehouse activity) (13). RTD for the perfect surveillance system would allow for “synchronous data” and RTD update, which is defined as a RTD architecture and RTD server. The next generation surveillance system must have RTD forecasting and analysis with thresholds (predefined “panic value”), duplicate data certification, and transmission logging of alarms, recommendations, and actions for appropriate agencies (i.e., telephone, pagers, e-mail lists, and data-synchronized client alarms). The ability to respond dynamically to specific detection information is an example of the capability to differentiate “signal” from “noise.” This type of system will incrementally collect better information about high-risk populations and reduce the burden of time and effort by first responders and health care providers.
PREPAREDNESS SUMMARY
From news reports, federal, DoD, and state agency reports, there are hundreds of parallel health surveillance systems being developed. The National Association of County and City Health Officials is funding more than $400 million of separate public health surveillance and training programs (14).
Jerry Hauer, a senior advisor to the Secretary for the Department of Health and Human Services for national security and emergency management, said in the proceedings of the Department of Health and Human Services National Committee on Vital and Health Statistics meeting held in February 2002, “I think one of the greatest concerns we have is that as different systems have been developed, be it syndromic or data mining, there has not been a commonality of data that is required. We will wind up with a group of systems around the country that don’t necessarily talk with one another” (15). Hauer is absolutely correct in saying that no surveillance system can be realized when confronted with our current medical detection coding methodology. Effective health and environmental standardized data detection information for data mining and syndromic surveillance function is not an option, it is a critical requirement.
A discussion of the Framingham Heart Study, one of the greatest modern research programs, illustrates this point clearly. Before 1950, it was believed coronary disease was unavoidable and people could not change their risk because the risk factors for disease surveillance were not understood. To determine what constellation of factors from the biological, environmental, and living style spheres could predict risk for myocardial infarction was one-half of the researchers’ objective. The other half was the novel concept that physicians and their patients take action and prevent what was thought to be inevitable. The detection of high blood pressure, serum cholesterol, age, dietary habits, and exercise habits were not incorporated into a surveillance program for heart disease until the results of this study changed risk behavior in the United States forever. However, the final results of this direct syndromic surveillance study took decades to make its predictions and be validated in subsequent trials. The value of standardized medical data to deliver medical care information more effectively and collect detection information without error will also provide the key to early identification of disease trends and the ability to make strategic decisions to protect the health of the population.
The U.S. military developed an innovative health care strategy called Force Health Protection (FHP), which uses preventive health techniques and emerging technologies in environmental surveillance and combat medicine to protect service members before, during, and after deployment. The overarching goal of FHP is casualty prevention, achieved through a physically and mentally fit force trained for modern combat. FHP prevention concentrates on environmental and health hazards and preventive strategies to reduce the largest number of military casualties caused by disease and nonbattle injury (DNBI) (16).
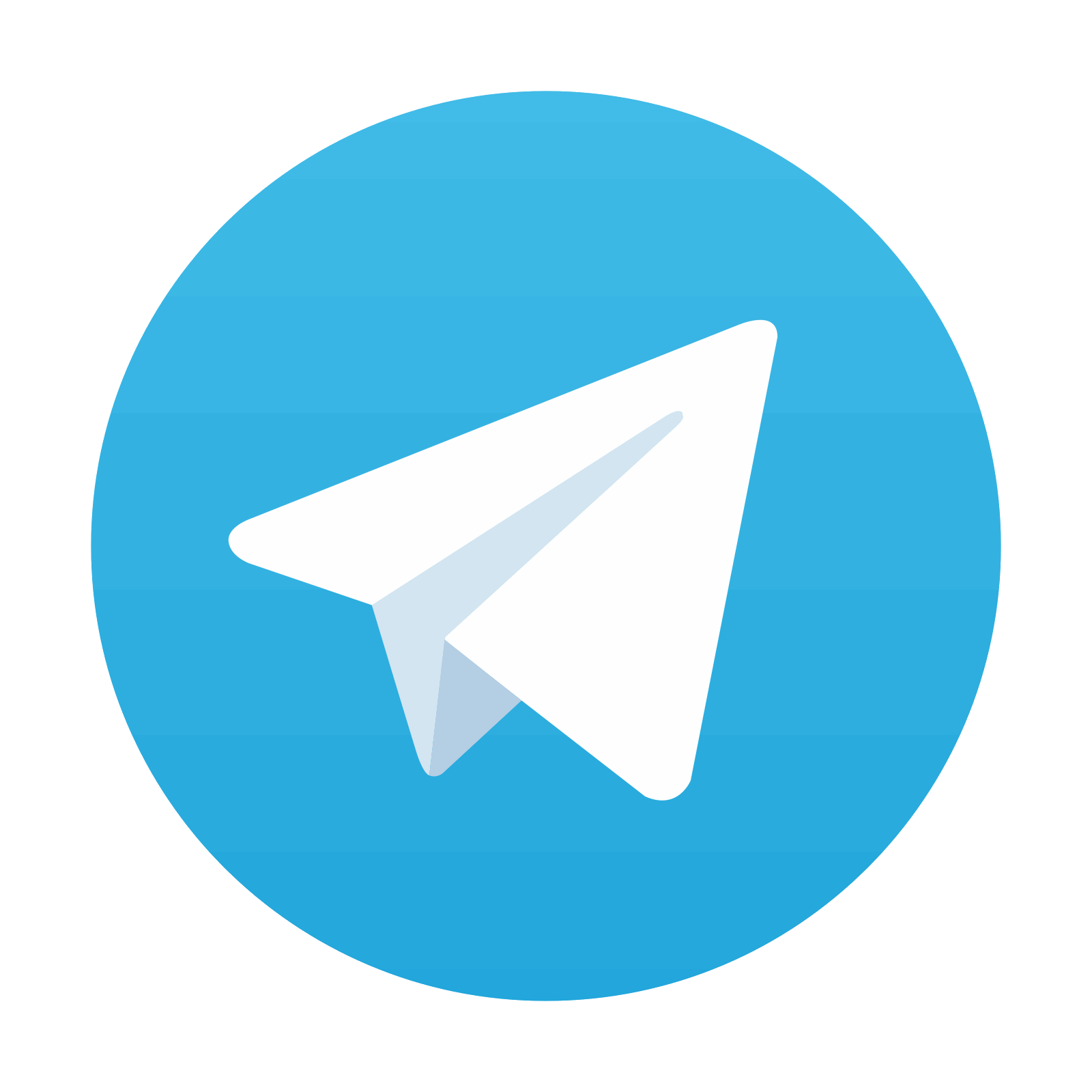
Stay updated, free articles. Join our Telegram channel

Full access? Get Clinical Tree
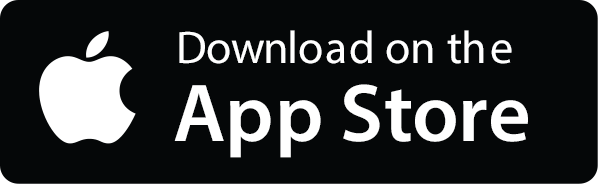
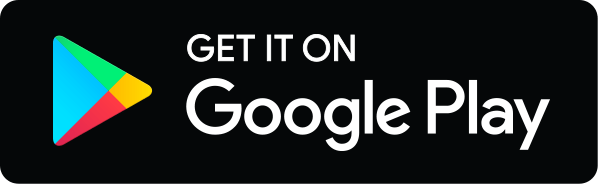