Detection of Nuclear Radiation
Keith L. Edsall
Daniel C. Keyes
INTRODUCTION
Ionizing radiation is not detectable by any natural means. Specific devices are necessary to detect alpha, beta, gamma, x-rays, and neutrons. Medical staff must learn to use simple portable instruments and interpret the findings, and they must also be aware of the associated pitfalls when using these instruments. Understanding the technical aspects of the working of the instrument is not essential, but those interested can obtain the information from any nuclear physics textbook. Note that this chapter uses the units of rads and rems, currently used in the United States. See Chapter 13 for a discussion of newer international units of measurement used in discussing radiation.
RADIATION DETECTION INSTRUMENTS
Instruments used to detect radiation can be divided into three major categories: portable instruments, laboratory instruments, and personnel and area dosimeters (Table 14-1).
TABLE 14-1 Instrument Requirements Based on the Type of Radiation Incident | |||||||||||||||||||||||||||
---|---|---|---|---|---|---|---|---|---|---|---|---|---|---|---|---|---|---|---|---|---|---|---|---|---|---|---|
|
PORTABLE INSTRUMENTS
The portable instruments that hospital and prehospital personnel use are capable of detecting contamination on the patient and also the dose rate coming off the patient as a result of external or internal contamination. Many medical decisions can be made from the information gained.
The Geiger-Müller “Counter”
The most common type of portable instrument used is the Geiger Müller, known familiarly as the “Geiger counter.” It is constructed using either an end window probe to detect beta and gamma radiation or a pancake probe to detect alpha, beta, and gamma radiation (Fig. 14-1).
Chapter 13 describes the penetrating characteristics of the different types of radiation and how to provide protection. Alpha radiation can be completely shielded by a simple piece of paper; beta particle shielding can be achieved by using a light material such as aluminum. Gamma radiation can only be attenuated by using heavy materials such as lead, depleted uranium, or blocks of concrete; and finally, neutrons are shielded by materials with a high hydrogen content such as polystyrene or wax.
The Geiger counter detects beta and gamma irradiations using the end window device. With the window facing the contamination, two types of radiation can be detected, beta and gamma. By turning the end window 90 degrees, the casing of the end window probe, which is made of metal, only permits gamma radiation through, shielding out beta radiation. Therefore the types of radiation can be differentiated.
The Pancake Probe
The typical pancake probe, more sensitive than the end window, is capable of detecting alpha, beta, and gamma radiations. However, it is inefficient when it comes to detecting alpha radiation. Special probes are used with a thin Mylar film cover containing zinc sulphide. Scintillation is used to measure alpha radiation, requiring a scintillation material known as a phosphor. When a phosphor material is exposed to ionizing radiation, it causes excess energy due to electron excitation and produces a flash of light. These flashes of light are directed onto a photo multiplier tube that changes them into electrical pulses, and by means of electronic circuits is able to record readings on an instrument scale.
The scintillation counter’s advantages over a Geiger-Müller instrument are that it has greater range for measuring dose rate, is capable of measuring higher count rates, and demonstrates improved accuracy when measuring dose rates. Removing the protective cover from the pancake probe, one can detect the three types of radiation, which can be differentiated by using simple shielding.
Placing a piece of paper between the radiation source and the probe of the instrument shields out alpha, allowing only the beta and gamma radiation to reach the probe of the instrument. Placing a thin piece of aluminum between the radiation source shields out beta radiation and also alpha radiation, allowing only gamma to reach the probe. The pancake probe casing is generally made out of aluminum. By turning the head 180 degrees, the beta radiation is shielded out. Therefore users have a good idea of what types of radiation they are dealing with and what radiation is the main contributor.
The Geiger-Müller instrument can be used to measure counts per minute or dose rate in mR/Hr. 1 mR/Hr corresponds to approximately 2,000 to 3,000 counts per minute. A word of caution: These instruments only detect low levels of gamma, and they can saturate with high-count levels giving a zero reading. Typical range for a Geiger-Müller counter is up to 80,000 counts per minute and up to 20 m/R per hour. Note that these instruments are calibrated against a known gamma source. For higher levels of gamma, a different type of instrument is required (e.g., an ionizing chamber, which can read levels up to 500R/Hr), and assistance should be obtained from a nuclear health physicist.
The Geiger-Müller instrument only distinguishes types of radiation, but it does not indicate what specific isotope or isotopes are causing the radiation. Identification of isotopes can only be achieved by using specialized laboratory techniques such as a mass spectrometer. Thus contaminated clothing, wound dressings, and other related samples should be sent as soon as possible to the designated nuclear laboratory for identification of radioactive isotope/isotopes.
Generally speaking, these types of instruments have a low sensitivity, in the range of 10%. Therefore only part of the irradiation/contamination present is being measured.
BACKGROUND RADIATION AND INTERPRETATION OF DETECTION DEVICES
We live in a “radiation environment.” The annual background radiation and human-made radiation in the United States is estimated as 360 m/rem. The contributions are 87% natural occurring, approximately 11.5% medical, 0.5% weapon fallout, 0.5% air travel and luminous dials, and 0.5 % occupational exposure.
We are continuously exposed to natural occurring sources including cosmic radiation, terrestrial gamma rays, internal radiation, and decaying products such as radon. We also have internal radiation: We are all “radioactive” with carbon 14 (C14) and potassium 40 in our bodies in very small amounts. C14 is produced by cosmic radiation interacting with nitrogen in the upper atmosphere changing N14 to C14. The C14 diffuses down into the lower atmosphere where it is absorbed by live tissue, and radioactive potassium 40 is found in the soil.
We are also exposed to human-made radiation, especially from medical sources including x-rays, nuclear medicine procedures, and radiotherapy procedures. Nuclear weapon tests also contribute to background exposure, but this is declining since the cessation of atmospheric testing in the mid-1960s. Other trivial contributions come from the nuclear industry.
It is important to know the level of background radiation in your area. Background radiation varies from area to area and from day to day. If you live in a mountainous region, the background radiation is much higher than in a “sedimentary,” or low-altitude region. Measure the background radiation in your area regularly so that in a radiation incident you will be able to calculate the increase above this background level. There have been cases in which it was erroneously assumed some individuals were contaminated, when in fact it was background radiation being measured. In areas of low natural radiation, the background count might be approximately 20 to 30 counts per minute. In areas with greater natural background levels, the count would be much higher. Therefore it is essential you measure the background for that particular day, especially before patients arrive at your hospital. You are looking for any increase in the instrument count level above background.
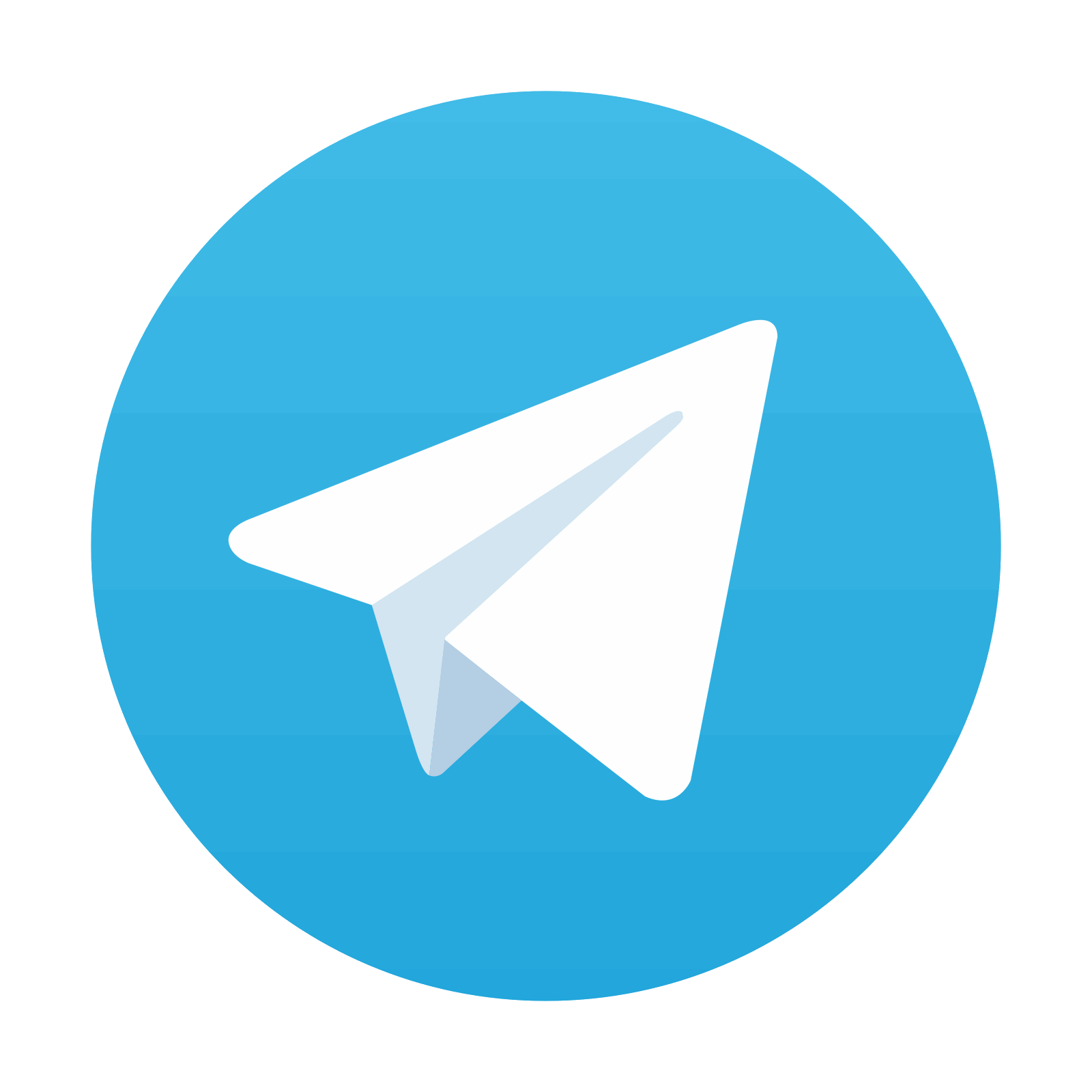
Stay updated, free articles. Join our Telegram channel

Full access? Get Clinical Tree
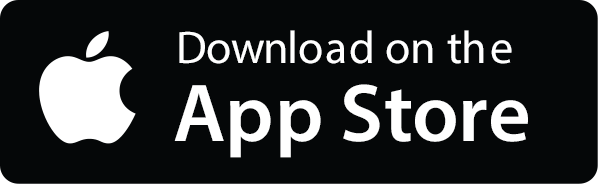
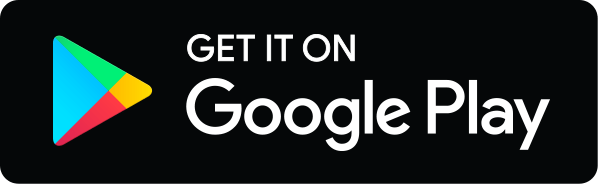