COVID-19 Vaccines
Gabriel Dagotto
Catherine Jacob-Dolan
Dan H. Barouch
INTRODUCTION
Vaccines get their name from the Latin word for cow, vacca, in part because the first vaccine, introduced by Edward Jenner in the 1700s to prevent smallpox, was based on cowpox.1 Cowpox (vaccinia virus) infects humans without causing severe disease but is antigenically similar enough to smallpox that vaccinated individuals are protected against smallpox.1,2
Since the discovery of vaccinia, the field of vaccinology has progressed. The advent of molecular biology techniques allowed for the isolation and study of specific pathogens and the rational design of vaccines.2,3 Modern vaccines are most often based on one of two rational designs: the delivery of an inactivated or attenuated form of the pathogen or the delivery of a key antigen from the pathogen.3, 4, 5, 6, 7, 8, 9, 10, 11, 12, 13 and 14 In both cases, the vaccine aims to induce protective immunity in the individual in the form of adaptive immune memory, which can either prevent or rapidly control infection upon exposure to the pathogen, thereby preventing severe disease.3,14,15 Vaccines are designed to deliver relevant antigens that induce robust immune responses, including durable immunologic memory to the antigen.3, 4, 5, 6, 7, 8, 9, 10, 11, 12, 13 and 14
Antigens are typically proteins from the pathogen in question and can be delivered via various vaccine platform technologies. A vaccine may also contain an adjuvant, which enhances innate immunity.14,16 Innate immune cells, including activated antigen-presenting cells (APCs), sense and take up the vaccine antigen. After trafficking to the local draining lymph node, APCs present the antigen alongside costimulatory signals to adaptive immune cells such as B and T cells, activating those cells that are specific for the antigen.14,17
Vaccine-elicited B and T cells then differentiate into effector cells, which contribute to clearance of the pathogen, and memory cells, some of which reside near the site of vaccination as tissue-resident memory cells and some of which circulate throughout the entire body as central memory cells, which are poised to respond rapidly upon antigen reexposure.14,18, 19 and 20 Should the same antigen be encountered again, either because of a second vaccination or exposure to the pathogen itself, these memory cells will expand rapidly and participate in an anamnestic adaptive immune response that is more rapid and more potent than the primary adaptive immune response.14,15,21
Most, though not all, current coronavirus disease 2019 (COVID-19) vaccines function by delivering the spike protein of severe acute respiratory syndrome coronavirus 2 (SARS-CoV-2).22, 23, 24 and 25 This antigen elicits humoral and, in most cases, also cellular immune responses to the spike protein, which protect the vaccinated individual from infection and/or disease.22, 23, 24, 25, 26, 27, 28, 29, 30, 31, 32 and 33 Antibodies against spike fall into two main classes: neutralizing and non-neutralizing. Neutralizing antibodies bind spike and prevent binding to the angiotensin-converting enzyme 2 (ACE-2) receptor on host cells and thus prevent infection.34, 35, 36 and 37 Non-neutralizing antibodies bind spike typically without blocking ACE-2 binding and induce other protective functions, such as inducing cellular responses to infected cells or activating complement.37,38 Neutralizing antibodies can perform these functions as well.35,37,38 T cells specific for spike support the development of B cells that are required for antibody production (CD4+ follicular helper T cells [Tfh cells]), coordinate the overall immune response including heightening innate immune surveillance to decrease infectivity of nearby stromal cells (CD4+ T helper type 1 cells [Th1 cells]), and exert direct antiviral activity by clearing infected cells, thus controlling virus dissemination (CD8+ cytotoxic T lymphocytes [CTLs]).39, 40, 41, 42, 43 and 44
TRADITIONAL VACCINE PLATFORM TECHNOLOGIES
Before reviewing specific details of current COVID-19 vaccines, we first describe current vaccine platforms and how they have been used in the past (Figure 17.1). The main vaccine platforms that were used in humans prior to the COVID-19 pandemic were live attenuated vaccines, inactivated vaccines, protein-based vaccines, and early use of viral vectored vaccines, such as for Ebola.14
Live Attenuated Vaccines
Live attenuated vaccines contain, as their name implies, a live replicating pathogen that is no longer highly pathogenic. Examples of live attenuated vaccines include vaccinia virus for smallpox, the Sabin oral polio vaccine (OPV), the yellow fever vaccine, and the measles/mumps/rubella (MMR) vaccine.45,46 In each of these instances, the pathogenic virus was modified or substituted with a less pathogenic version of the virus. The weakened version of the virus is still able to infect the vaccine recipient and triggers an immune response that reacts against the original pathogenic virus.45 The attenuated vaccine virus also triggers innate immune signaling cascades and induces robust adaptive immune responses similar to the actual infection.45 Live attenuated vaccines are known to induce some of the most robust and durable humoral and cellular immune responses, including both CD8+ and CD4+ T-cell responses.14 However, there are risks with live attenuated vaccines, and immunocompromised individuals may not be able to clear the vaccine virus, which could result in virulence.45
Inactivated Vaccines
An inactivated vaccine consists of the original pathogen that has been rendered inert via chemical and/or physical disruption.47 The Salk inactivated polio vaccine (IPV) and the seasonal influenza vaccine are examples of inactivated virus vaccines.46,47 Although inactivated virus vaccines are generally safer than attenuated virus vaccines, they may have lower immunogenicity as a result of the inactivation process.46,48 Additionally, inactivated viruses do not productively infect cells in the vaccinated individual, sometimes
necessitating the addition of an adjuvant.49 Inactivated virus vaccines can induce robust humoral responses but often fail to induce strong CD8+ T-cell responses, as compared to live attenuated vaccines.14
necessitating the addition of an adjuvant.49 Inactivated virus vaccines can induce robust humoral responses but often fail to induce strong CD8+ T-cell responses, as compared to live attenuated vaccines.14
Protein Subunit Vaccines
As molecular biology techniques advanced, it became possible to deliver antigens independently from the pathogen of origin. Many vaccines deliver protein antigens without the rest of the original pathogen, which enhances safety. Some vaccines, such as those against diphtheria and tetanus, present inactivated versions of the toxin proteins (toxoids) made by the pathogens. The Tdap vaccine contains tetanus toxoid, diphtheria toxoid, and proteins from pertussis.14 This vaccine protects against all three pathogens by delivering key proteins allowing the immune system that triggers protective antibody responses.50 Other protein-based vaccines include human papillomavirus (HPV), hepatitis A virus (HAV), and hepatitis B virus (HBV).14 These vaccines deliver key antigens from the viruses in question, often in the context of a multivalent virus-like particle.50 Protein subunit vaccines often include an adjuvant to stimulate the innate immune system and enhance adaptive immune responses.50 Protein subunit vaccines induce strong humoral responses but do not typically induce CD8+ T-cell responses.14
NOVEL VACCINE PLATFORM TECHNOLOGIES
Recent advances in vaccine technology have facilitated the development of viral vector and nucleic acid vaccine platform technologies (Figure 17.1). These platforms allow faster development compared with traditional inactivated and live attenuated vaccines, and they do not require the actual pathogen for vaccine development.51 These gene-based vaccines focus the immune system on only the key viral immunogen(s) while avoiding the rest of the pathogen. Adenoviral vector vaccines, DNA vaccines, and messenger RNA (mRNA) vaccines all are self-adjuvanted and thus do not require additional adjuvants in most situations.24,25,52
For SARS-CoV-2 vaccines, previous work on other CoV vaccines (SARS, Middle East respiratory syndrome [MERS]) identified the spike protein as the primary vaccine target.23,53 Previous work also identified a diproline mutation in the S2 domain to stabilize the spike in its prefusion conformation, which has been incorporated into most SARS-CoV-2 vaccines.54 Additionally, the spike has a furin cleavage site that is necessary for virus entry into cells, and some SARS-CoV-2 vaccines have also deleted the furin cleavage site.55
Adenoviral Vectors
Adenoviruses were first identified as tissue culture cytopathic agents isolated from adenoid tissue in the 1950s. These infectious particles were determined to be viruses after filtration did not prevent infection, and attempts to grow the agent on bacterial media failed.56 After adenoviruses were further studied, they were identified as viruses capable of causing the common cold and other clinical syndromes in people.
Currently, there are over 100 known adenovirus serotypes.57,58 The Adenoviridae family is comprised of approximately 36 kb linear, double-stranded DNA (dsDNA) viruses with a typical diameter of around 70 to 100 nm.59 The viral capsid is icosahedral with fibers that extend outward and allow the virus to make contact with its cell surface receptors.60 Cell entry receptors vary based on the exact virus but the most commonly used receptors include the coxsackievirus and adenovirus receptor (CAR), CD46, and sialic acid.61, 62, 63 and 64 Adenoviruses enter host cells through endosomes and then traffic to the nucleus where transcription and replication occur.65 The adenovirus genome mainly consists of four early open reading frames (ORFs; E1, E2, E3, E4) and five late ORFs (L1, L2, L3, L4, L5).
Adenoviruses were converted into replication-incompetent adenoviral vectors (Ad vectors) by removing E1, and in some cases E3, and inserting a transgene cassette.66 These vectors are attractive vaccine platforms as they are easy to grow and manipulate and they have high stability, broad cellular tropism, no genome integration, robust immunogenicity including both humoral and cellular immune responses, and a long track record of safety in clinical trials.25,67,68
Adenoviral vectors consist of a capsid primarily composed of the hexon, penton, fiber, and multiple minor proteins encasing a dsDNA genome encoding portions of the adenoviral genome along with a transgene cassette containing the immune antigen of interest.69 The first-generation Ad vectors were designed as gene therapy vectors70 to treat diseases such as cystic fibrosis,71 α-1 antitrypsin deficiency,72 and ornithine transcarbamylase deficiency.73 In these studies, and later human trials, although the Ad vectors were able to express the target gene, there was also a potent immune response against the Ad vector and the transgene, making gene therapy less effective.66,74,75 The high immunogenicity of Ad vectors limits their potential for gene therapy but makes them attractive for vaccine development.
Ad vectors generate strong immune responses by multiple mechanisms.76 Adenoviral vectors include pathogen-associated molecular patterns (PAMPs) that trigger Toll-like receptor (TLR) signaling through TLR2, TLR4, and TLR9.77, 78 and 79 The ability to engage multiple TLRs is thought to contribute to the potent immunogenicity of Ad vectors.76 The viral DNA also triggers innate immunity through the cGAS/STING pathway.80 Ad vectors have also been shown to trigger the NLRP3 inflammasome.81 Activation of these innate signaling pathways occurs with Ad vectors as well as infectious Ad virus. Some innate immune activation is thought to be required for Ad vector immunogenicity, but too much activation may also be detrimental.82,83 Some strategies to reduce innate immunity may lead to more prolonged transgene expression.76 Ad vectors generate robust humoral immunity and are particularly good at inducing high CD8+ T-cell responses.84,85
Adenovirus serotype 5 (Ad5) was originally the most commonly used Ad vector, but recently other Ad serotypes have been utilized because of high levels of baseline immunity against Ad5 (and other
species C Ads) in human populations.86 Ad5 still has a prominent role in the field,87 but alternative human serotype Ad vectors and Ad vectors from macaques,88,89 chimpanzees,90 other nonhuman primates,91 and other species92 are available. Currently, Ad vectors are involved in over 200 clinical trials, and Ad vector–based vaccines have been developed against Zika virus and Ebola virus.67,68 Four Ad vector– based vaccines have been developed against SARS-CoV-2 for global use.32,90,93 The Ad26-based vaccine from Janssen/J&J is the only single-shot vaccine currently available, although a second shot increases immunogenicity and efficacy.32 Although the Ad26 vaccine induces lower peak antibody titers compared with mRNA vaccines, the durability of the Ad26 vaccine appears substantially better than mRNA vaccines.22 Additionally, as new variants emerge with progressively increasing resistance to neutralizing antibodies, the relevance of the robust cross-reactive CD8+ T-cell responses induced by Ad vectors may also increase.
species C Ads) in human populations.86 Ad5 still has a prominent role in the field,87 but alternative human serotype Ad vectors and Ad vectors from macaques,88,89 chimpanzees,90 other nonhuman primates,91 and other species92 are available. Currently, Ad vectors are involved in over 200 clinical trials, and Ad vector–based vaccines have been developed against Zika virus and Ebola virus.67,68 Four Ad vector– based vaccines have been developed against SARS-CoV-2 for global use.32,90,93 The Ad26-based vaccine from Janssen/J&J is the only single-shot vaccine currently available, although a second shot increases immunogenicity and efficacy.32 Although the Ad26 vaccine induces lower peak antibody titers compared with mRNA vaccines, the durability of the Ad26 vaccine appears substantially better than mRNA vaccines.22 Additionally, as new variants emerge with progressively increasing resistance to neutralizing antibodies, the relevance of the robust cross-reactive CD8+ T-cell responses induced by Ad vectors may also increase.
DNA Vaccines
DNA vaccines consist of a plasmid dsDNA encoding the antigen of interest under a mammalian promoter.52 In the 1990s, naked DNA was shown to be capable of transducing mouse cells and producing a protein after intramuscular (IM) injection.94 This work showed that DNA vaccines did not have to be packaged or formulated in complex lipids to transduce cells. Studies then showed that DNA vaccines were effective against influenza in mice.95 DNA vaccines provided benefits such as flexibility of production, high purity, nonreplicating leading to increased safety, negligible risk of genome integration,96 no vector-specific immunity, and relatively long antigen expression in tissues.
DNA vaccines enter cells and trigger the innate immune system by activating TLR9 because of CpG motifs in the plasmid DNA.97,98 TLR9 activation is believed to contribute to DNA vaccine immunogenicity, but experiments with TLR9 knockout mice have shown that it is not essential for DNA vaccines.99 The double-stranded structure of the DNA vaccines also stimulates innate immunity through noncanonical IKK and TANK-binding kinase-1 (TBK1)-dependent innate immune pathways.100,101 Additionally, DNA vaccines stimulate the cGAS-STING pathway,102, 103 and 104 AIM2,105 and other DNA sensors.106 Once inside the cell, DNA vaccines produce the encoded transgene, which is then presented by APCs in the draining lymph node, resulting in antibody responses as well as CD4+ and CD8+ T cells.
DNA vaccines have been shown to induce both humoral and cellular immune responses following vaccination.107 DNA vaccines have demonstrated remarkable success in multiple animal models,106,108 but the strong immunogenicity of DNA vaccines observed in mice was not observed in humans.52 The greatest limitation to DNA vaccines is suboptimal immunogenicity in humans.106 There are no clinically approved DNA vaccines, but DNA vaccines have been used as research tools for screening SARS-CoV-2 antigens in preclinical studies.55
mRNA Vaccines
mRNA vaccines have recently emerged as an important novel vaccine platform. mRNA vaccines were shown to induce protein expression in animals in 1990 along with DNA vaccines.94 Development of mRNA vaccines was initially slow because of difficulties with instability, vulnerability to RNAses, undesirable immunogenicity, and manufacturing problems.24 Many of these issues have been resolved with codon optimization,109 modified nucleotides to reduce undesirable inflammatory responses,110,111 and high-performance liquid chromatography to increase mRNA purity.112 Of particular importance has been the development of lipid nanoparticles for mRNA vaccine delivery to address problems with instability. The most widely used mRNA vaccines are packaged into lipid nanoparticles consisting of four parts: PEGylated lipid, an ionizable lipid, cholesterol, and a helper lipid.113 mRNA vaccines have become particularly attractive vaccine candidates because of their remarkable potency for inducing high antibody titers, ease and speed of production, noninfectious and nonintegrating nature, and no vector-specific immunity. These features led to multiple mRNA vaccine candidates for SARS-CoV-2, and the Pfizer BNT162b2 and Moderna mRNA-1273 vaccines having been approved and deployed throughout the world.26,114
mRNA vaccines are taken up by APCs through endocytic pathways24,115 and trigger innate immunity through pattern recognition receptors (PRRs) such as TLRs (TLR3, TLR7, TLR8), MDA5, RIG-I, and NOD-like receptors. These innate immune responses lead to the production of type
I interferons. Similar to viral vectors, interferons may have both positive and negative impacts on vaccine immunogenicity. Type I interferons can activate APCs and increase B- and T-cell responses but can also limit transgene expression.24 Recent work has suggested that sustained antigen expression is critical for durable antibody responses and germinal center B- and T-cell responses.116 The use of modified base pairs such as N1-methyl-pseudouridine minimizes innate immune responses to mRNA vaccines by reducing TLR7/8 activation, thus allowing higher levels of antigen expression and reduced reactogenicity that allows higher vaccine doses.117 mRNA vaccines induce high antibody titers as well as moderate levels of T-cell responses.118 Current areas of research include optimization of untranslated region (UTR) modifications, base pair alterations, and delivery methods.119
I interferons. Similar to viral vectors, interferons may have both positive and negative impacts on vaccine immunogenicity. Type I interferons can activate APCs and increase B- and T-cell responses but can also limit transgene expression.24 Recent work has suggested that sustained antigen expression is critical for durable antibody responses and germinal center B- and T-cell responses.116 The use of modified base pairs such as N1-methyl-pseudouridine minimizes innate immune responses to mRNA vaccines by reducing TLR7/8 activation, thus allowing higher levels of antigen expression and reduced reactogenicity that allows higher vaccine doses.117 mRNA vaccines induce high antibody titers as well as moderate levels of T-cell responses.118 Current areas of research include optimization of untranslated region (UTR) modifications, base pair alterations, and delivery methods.119
Over the past several years, mRNA vaccines have evolved from a novel vaccine platform to several clinically approved vaccines and dozens in clinical trials. The approved mRNA vaccines for SARS-CoV-2 include BNT162b2 (Pfizer/BioNTech) and mRNA-1273 (Moderna).26,114 Both vaccines express the modified SARS-CoV-2 spike protein and require a two-shot primary regimen and boosters. The initial phase 3 clinical trials showed outstanding efficacy at preventing symptomatic COVID-19 prior to the emergence of viral variants with a follow-up period of 63 days26 and 2 months, respectively.31 However, subsequent studies showed that the durability of these mRNA vaccines is limited, with antibody titers and protective efficacy declining after 3 months and even further after 8 months,120, 121, 122 and 123 and also reduced by the emergence of SARS-CoV-2 variants.
ADJUVANTS, INNATE IMMUNITY, AND ADAPTIVE IMMUNITY
Vaccines need to deliver both the target antigen and the appropriate danger signals that augment the immune response against it (Figures 17.2 and 17.3).14,50 This second component, known as an adjuvant, can have a major impact on the nature and magnitude of the induced immune response and the protective efficacy of the vaccine.124 Some vaccines are “self-adjuvanting,” which means that within the vaccine itself are molecules that trigger innate immune recognition. Live attenuated viruses, for instance, contain all the PAMPs of a live virus and therefore trigger a variety of PRRs in innate immune and stromal cells.45 Many inactivated virus vaccines include PAMPs from the virus as well.46 Though the virus might no longer be infectious, delivery of the protein antigens with viral nucleic acids may be sufficient to trigger innate antiviral responses.46
Some vaccines, however, are not self-adjuvanting. For example, protein subunit vaccines contain only specific protein immunogens and thus often require an additional adjuvant.50 Immune stimulatory molecules must therefore be added to ensure the vaccine elicits the desired response. Historically, adjuvants such as alum salts have been used. When mixed with alum, protein subunit vaccines can induce higher antibody titers, although CD8+ T-cell responses are typically not induced by alum adjuvanted protein vaccines.50,125,126
Over the past several years, the science of adjuvants has advanced. Molecules that specifically target different PRRs such as TLR7 or TLR9 have been leveraged to influence and expand immune response to vaccines, inducing a more Th1 biased immune response.16,50,127,128 Other adjuvants include oil-in-water emulsions, such as MF59 or AS01B.16,50,127 These adjuvants have been shown to augment humoral and cellular immune responses to protein subunit vaccines, even with limited doses of protein. These oil-in-water emulsions induce transient immune activation at the site of injection, which promotes cytokine and chemokine production and recruits APCs to the site of immunization,128 resulting in enhanced activation and antigen uptake and amplification of adaptive immune responses.128
Adenoviral and mRNA vaccines are self-adjuvanting and typically do not require additional adjuvants. For mRNA vaccines, the lipid nanoparticle is immunostimulatory and essentially functions as an adjuvant.129,130 Additionally, the mRNA itself has adjuvant properties, depending on the nature of the RNA backbone modifications.131 Moreover, the immunogenicity of mRNA vaccines can be modulated by modification of the 5′ and 3′ UTRs.10 This allows tuning of both innate and adaptive immune responses, as well as ensuring sufficient translation of the antigen of interest.10,12,132 Insufficient protein translation would lead to a suboptimal response, but excessive translation could be tolerogenic.7,10,12,131, 132 and 133 For adenoviral vectored vaccines, multiple different innate sensors recognize the viral genome of the adenovirus vector.134, 135 and 136 Additional innate immune stimulation may come
from the capsid proteins, although empty adenoviral capsids induce far weaker innate immunity compared with adenoviral capsids containing viral DNA.135,136 Engineering of the adenoviral capsid represents a strategy to modulate vector immunogenicity.
from the capsid proteins, although empty adenoviral capsids induce far weaker innate immunity compared with adenoviral capsids containing viral DNA.135,136 Engineering of the adenoviral capsid represents a strategy to modulate vector immunogenicity.
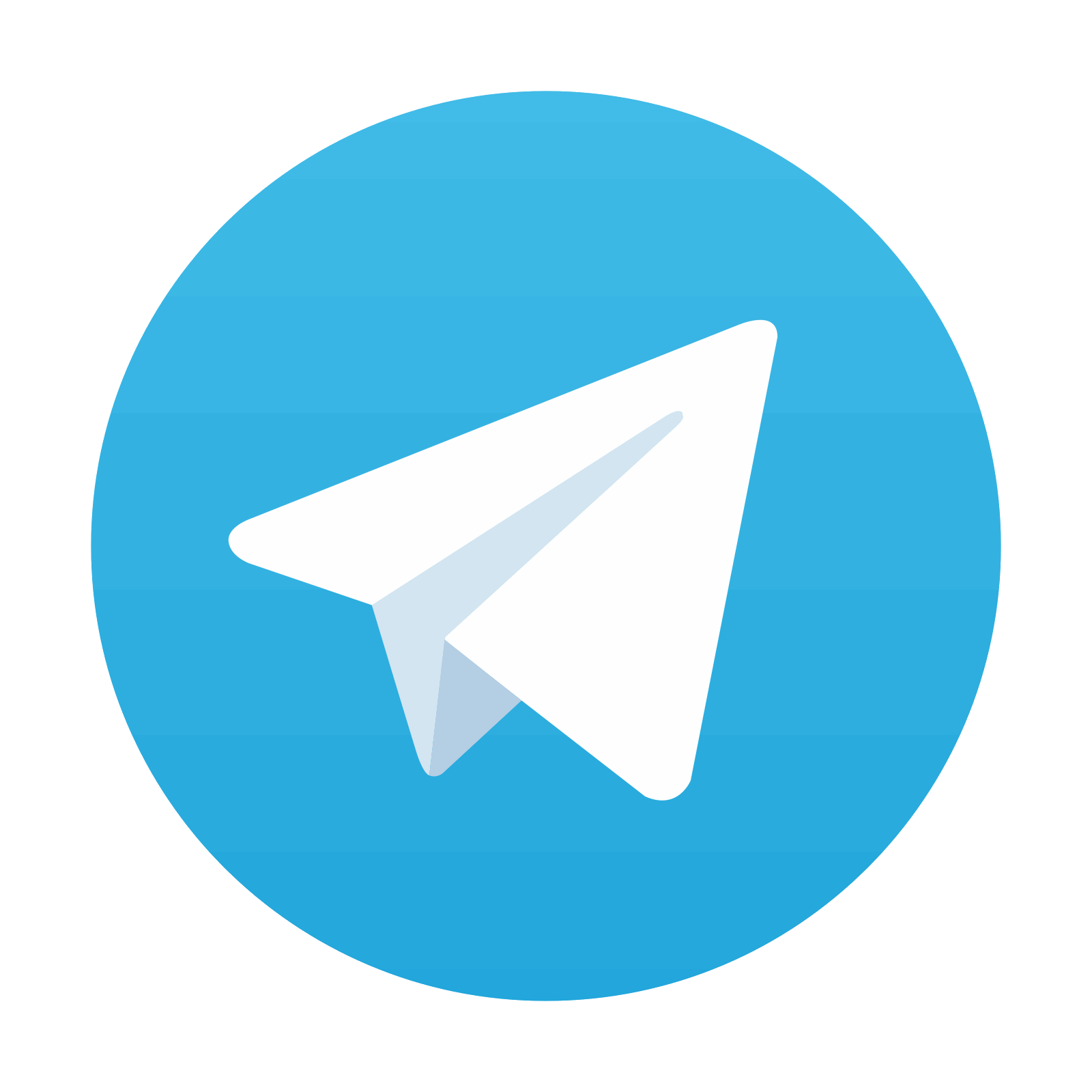
Stay updated, free articles. Join our Telegram channel

Full access? Get Clinical Tree
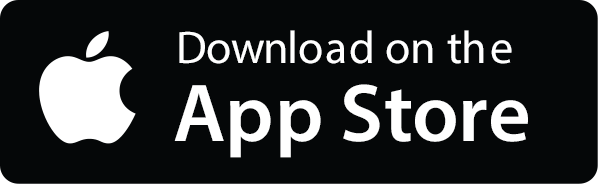
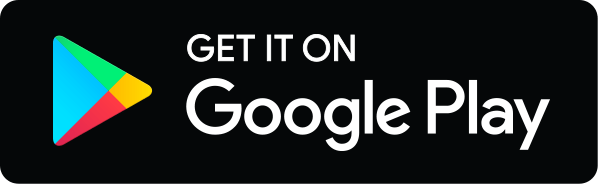