COVID-19 Testing for Medicine and Public Health
Michael J. Mina
INTRODUCTION
The coronavirus disease 2019 (COVID-19) pandemic has caused unprecedented tragedy and disruptions to society on a global scale. When the severe acute respiratory syndrome coronavirus 2 (SARS-CoV-2) virus began to spread, testing for the virus was one of the first and most critical processes to establish.1 Testing for a human virus pathogen like SARS-CoV-2 is usually considered a medical procedure, but as the public health emergency associated with this virus demonstrated, testing isn’t just for medical purposes. In the context of a global pandemic, testing is how we see the virus. It is our eyes on the virus. Without testing during a pandemic, public health officials and policymakers through to each individual of society is left in the dark. Accurate, efficient, and scalable testing is thus one of the most important tools to develop early on to both prevent and limit the spread of a pandemic virus. However, the massive need for testing during a pandemic that spreads across the globe meant that testing would prove to be one of the most formidable challenges throughout the pandemic.
COVID-19 testing is a vital tool in the public health arsenal for identifying infected individuals, isolating them, and mitigating the spread of the virus.2 Effective testing can enable the timely identification of new cases of COVID-19 and facilitate necessary contact tracing, isolation, and treatment to prevent further transmission. Knowing where the virus is, who it infects, and where it spreads most efficiently are each critical to allocate clinical and public health resources and develop public health strategies to stop or slow the spread. Testing is what provides the data to monitor and measure the pandemic virus across all scales from individuals to whole populations.
When testing during a pandemic, it is essential to distinguish between medical diagnostic testing and public health testing and to ask the question, “What is this test being used for?” Medical diagnostic testing is employed to diagnose individual patients with COVID-19 symptoms, whereas public health testing is intended primarily to manage the spread of the virus and monitor prevalence at the population level. Although the testing tools used may overlap, the purpose of testing should dictate which type of test is going to be used.3
Medical diagnostic testing typically involves identifying individuals with symptoms of COVID-19, such as fever, cough, and shortness of breath. The principal tests usually used for diagnostic purposes for viruses like SARS-CoV-2 are nucleic acid amplification tests (NAATs), which include reverse transcription-polymerase chain reaction (RT-PCR) and loop-mediated isothermal amplification (LAMP) tests. These tests are highly specific and sensitive, and are considered the gold standard for diagnosing COVID-19. Antigen tests, which are very inexpensive, do not require any instrumentation and can be performed at the point of care. These have gained traction as critical tools for medical
diagnostics as well as public health tools during COVID-19. Antibody tests, which give a reading of past exposures to pathogens such as SARS-CoV-2, are critical tools in pandemic response; however, they are less useful for real-time medical diagnostic purposes. Antibody tests do, however, have a place in medicine, for example, to help identify when a booster vaccine may be required.
diagnostics as well as public health tools during COVID-19. Antibody tests, which give a reading of past exposures to pathogens such as SARS-CoV-2, are critical tools in pandemic response; however, they are less useful for real-time medical diagnostic purposes. Antibody tests do, however, have a place in medicine, for example, to help identify when a booster vaccine may be required.
Public health testing, on the other hand, encompasses broader testing of individuals and populations to prevent and slow transmission and guide public health approaches. Screening and surveillance testing are two types of public health testing that are employed to identify infected individuals, including asymptomatic individuals or those who do not have any symptoms. Screening testing is used to identify individuals who may have been exposed to the virus, such as healthcare workers or individuals living in congregate settings, whereas surveillance testing is used to monitor the spread of the virus in communities, including identifying individuals with no known exposure among the community. Antigen tests have become critical tools for public health screening and surveillance testing because of their rapid turnaround time, low cost, and access within the community.4,5
The COVID-19 pandemic and the devastating pressures and catastrophes it has placed on humans have driven new technologies to be developed to improve our ability to see the virus and respond, both from a medical and public health perspective. Testing and how it is used for pandemic response has changed dramatically since early 2020, particularly with the rise of rapid diagnostic tests. These advances have enabled faster and more accurate detection of
infections, providing critical information to public health officials to implement control measures and prevent the spread of the virus.6
infections, providing critical information to public health officials to implement control measures and prevent the spread of the virus.6
The COVID-19 pandemic has brought with it unique challenges in testing, including the need for rapid and accurate testing, the scarcity of resources, and the need for fast regulatory approval. The Emergency Use Authorization (EUA) process has been used to expedite the approval of COVID-19 tests, but at times had the opposite effect, particularly early in the pandemic, and hampered access to COVID-19 testing.7 The regulatory challenges have been particularly significant in the approval of rapid antigen tests. These tests have been developed to provide quick results and are less expensive than NAATs; however, they have lower sensitivity compared to NAATs, leading to delays in U.S. Food and Drug Administration (FDA) emergency clearance as medical devices.
In addition to diagnostic testing, monitoring of virus variants has been a crucial component of COVID-19 testing during the pandemic. The virus has mutated and evolved over time, resulting in new variants that may be more transmissible or more resistant to current treatments and vaccines. Next-generation sequencing (NGS) has been used to identify and track the evolution of the virus and detect new variants. Wastewater sequencing has also been employed to monitor the prevalence of the virus in communities, detect outbreaks early, and track the transmission of the virus.8,9
Looking to the future, new testing technologies are likely to have a significant impact on pandemic management. Digital health tools, such as contact tracing apps, may play a significant role in pandemic management by facilitating early detection and rapid response to outbreaks. The use of big data and artificial intelligence (AI) in pandemic management may also help public health officials to identify emerging trends, predict the spread of the virus, and make informed decisions about resource allocation and control measures.10,11
Despite the significant challenges posed by the COVID-19 pandemic, it has also brought about positive changes. The pandemic has spurred innovation and collaboration, leading to the development of new testing technologies and strategies. It has emphasized the importance of investing in public health infrastructure and working together to tackle public health challenges. The pandemic has also highlighted the resilience and adaptability of human societies in the face of adversity.
In the rest of this chapter, we will explore the various aspects of COVID-19 testing in greater depth. We will examine the different testing modalities, including their strengths and limitations, and how they are used in the COVID-19 pandemic. We will also discuss the regulatory issues related to COVID-19 testing, including the EUA process and the challenges of ensuring the quality and accuracy of tests. We will investigate the importance of monitoring virus variants through sequencing technologies and the role of wastewater sequencing in monitoring the spread of the virus and examine the challenges of comparing rapid antigen tests to PCR and the regulatory issues surrounding rapid antigen tests. Finally, we will explore the potential impact of new testing technologies on future pandemics, including digital health tools and AI.
COVID-19 testing is a vital tool in the public health response to the pandemic. Effective testing can help identify infected individuals, prevent transmission, and ultimately save lives. The development of new testing technologies and strategies has enabled faster and more accurate detection of infections, providing critical information to public health officials to implement control measures and prevent the spread of the virus. Although the COVID-19 pandemic has presented significant challenges, it has also spurred innovation and collaboration, leading to positive changes in public health infrastructure and management strategies.
PURPOSE OF TESTING IN A PANDEMIC
The purpose of testing during a pandemic is multifaceted and essential for understanding, controlling, and mitigating the spread of the disease. Primary goals of testing include identifying infected individuals in order to help limit transmission, understanding prevalence and transmission patterns of the virus, informing clinical care, and monitoring the effectiveness of public health interventions. This section discusses the goals of testing during a pandemic, including both diagnostic or medical testing as well as public health testing, and dives into the types of testing strategies that are employed for testing in a pandemic. This section also discusses some of the ethical concerns that should be considered while testing during a pandemic like COVID-19.
Diagnostic Testing
Diagnostic testing plays a critical role in managing the COVID-19 pandemic. The primary purpose of diagnostic testing is to identify individuals who are currently infected with the virus, allowing for appropriate clinical management, isolation, and contact tracing. This information is vital for guiding patient care, as well as informing public health strategies.
There are several types of diagnostic tests available, each designed to detect different components of the virus or the host’s immune response. These tests can be categorized as NAATs, antigen tests, and antibody tests.
NAATs, such as PCR, are designed to detect the viral genetic material, usually the RNA, in a patient’s sample. NAATs are highly sensitive and specific, making them the gold standard for diagnosing infections. However, they can be resource-intensive, require specialized laboratory equipment, and have a longer turnaround time for results compared to other diagnostic tests.
Antigen tests detect the presence of specific viral proteins, such as the coronavirus spike or nucleocapsid proteins, in a patient’s sample. These tests are generally faster and more straightforward to perform than NAATs, making them suitable for point-of-care testing. However, antigen tests may have lower sensitivity, particularly in the early and late stages of infection, which can result in a higher rate of false negatives.
Antibody tests, also known as serologic tests, detect the presence of antibodies generated by the host’s immune system in response to the virus. Although less useful for diagnosing active infections, antibody tests can help identify previous exposures to the virus and assess the immune response in individuals who have recovered from the infection. These tests can be valuable for understanding the prevalence of past infections in a population.
Not all tests are equally appropriate for diagnostic testing. Factors such as the stage of infection, the test’s sensitivity and specificity, the turnaround time for results, and the resources required for testing must be carefully considered when determining the most appropriate diagnostic test for a given situation.
Advantages of using diagnostic tests during a pandemic include identification of cases, which helps to limit the spread of the virus; the potential to inform treatment decisions, particularly for antiviral therapies and clinical trials; and guiding public health interventions such as isolation and contact tracing. However, trade-offs include the potential for false negatives or positives, logistic challenges in large-scale testing efforts, and the risk of overreliance on test results, which may lead to delays in implementing clinical measures.
Public Health Testing
Public health testing serves a different purpose from diagnostic testing, focusing on understanding and managing the pandemic and outbreaks on a population level.12 Public health testing can help identify asymptomatic and infectious carriers, assess the prevalence of the virus in different populations, monitor changes in transmission rates, and evaluate the effectiveness of public health interventions.
Types of public health tests include surveillance testing and screening, seroprevalence testing, and wastewater monitoring, among others. Surveillance testing involves monitoring the virus’s spread in a community by testing individuals regardless of their symptoms, risk factors, and many times
regardless of any known exposure. This type of testing is essential for identifying asymptomatic carriers, who can silently transmit the virus to others, and for calculating prevalence and incidence rates.
regardless of any known exposure. This type of testing is essential for identifying asymptomatic carriers, who can silently transmit the virus to others, and for calculating prevalence and incidence rates.
An essential aspect of public health surveillance testing is its role in isolating potentially infectious individuals to limit them from spreading to others and to initiate contact tracing, which is a vital strategy for controlling the spread of infectious diseases onward from secondary contacts. Contact tracing involves identifying individuals who have been in close contact with confirmed cases and monitoring them for signs of illness or testing them, for example, while in quarantine. In the context of COVID-19, testing is a critical component of contact tracing efforts. It allows for the rapid identification of infected individuals, including those who may be asymptomatic or presymptomatic. Promptly detecting and isolating new cases helps break chains of transmission and helps prevent further spread of the virus. Effective contact tracing requires robust testing infrastructure, efficient communication between public health authorities and the public, and a high degree of cooperation from individuals who may be asked to undergo testing, quarantine, or isolation.12,13
Seroprevalence testing estimates the proportion of individuals in a population who have been exposed to the virus, by assessing the presence of specific antibodies in blood or saliva that target the virus. This information can be valuable in understanding the extent of the pandemic and identifying areas with higher or lower exposure rates. Seroprevalence testing can also help estimate population-level immunity and inform vaccination strategies.14
Wastewater testing is a noninvasive method for monitoring the presence of the virus in a community. By analyzing wastewater samples for traces of the virus’s genetic material, public health officials can track changes in infection rates over time and identify potential outbreaks before they escalate. This approach can be particularly useful in areas where individual testing may be logistically challenging or resource-limited.9
Each of these public health testing methods has its advantages and disadvantages. Advantages of public health testing include the ability to understand and track the virus’s spread and transmission patterns, inform public health decision-making, and allocate resources effectively. These testing methods can also help evaluate the impact of public health interventions, such as lockdowns, social distancing measures, and vaccination campaigns, on the course of the pandemic.
But public health testing comes with challenges and disadvantages as well. The potential for sampling bias can arise if certain segments of the population are over- or underrepresented in testing efforts. This can harm efforts at allocating public health resources appropriately and lead to skewed estimates of prevalence and transmission rates as well as morbidity and mortality associated with infection. Additionally, interpreting results from different tests and comparing data across regions or countries can be challenging because of variations in testing strategies, methodologies, and reporting standards. Finally, large-scale public health testing efforts require significant resources, including personnel, equipment, and funding, which may be strained during a pandemic.
Both diagnostic and public health testing are essential tools in managing a pandemic. Diagnostic testing helps identify infected individuals, inform clinical care, and guide public health interventions at an individual level, whereas public health testing provides valuable population-level data on prevalence, transmission, and the effectiveness of public health measures. Balancing the advantages and disadvantages of different testing methods and strategies is crucial for optimizing pandemic response and ensuring the best outcomes for both individual patients and whole communities.
Sensitivity and Specificity
In the context of COVID-19 testing, sensitivity and specificity are critical measures that describe the performance and accuracy of a given test.
Sensitivity refers to the ability of a test to accurately identify individuals who have COVID-19. A test with high sensitivity is less likely to miss individuals who are infected. It is calculated as the number of true positives detected by the test, divided by the total number of actual positive samples under evaluation. The total number of actual positive samples under evaluation can be calculated by summing the number of samples that accurately result as positives plus the number of samples that should be positive but falsely result as negative, termed false negatives.
Sensitivity = True Positive/(True Positive + False Negative)
Specificity refers to the ability of a test to accurately identify individuals who do not have COVID-19. A test with high specificity is less likely to incorrectly identify individuals as infected
when they are not. Specificity is calculated by comparing the numbers of specimens accurately identified as negative to the total number of actual negative samples under evaluation. The total number of actual negative samples under evaluation can be calculated by summing the number of specimens that accurately result as negative plus the number of specimens that erroneously result as positive but are actually negative, termed false positives.
when they are not. Specificity is calculated by comparing the numbers of specimens accurately identified as negative to the total number of actual negative samples under evaluation. The total number of actual negative samples under evaluation can be calculated by summing the number of specimens that accurately result as negative plus the number of specimens that erroneously result as positive but are actually negative, termed false positives.
Specificity = True Negative/(True Negative + False Positive)
In medical and public health testing, understanding a test’s sensitivity and specificity is crucial for appropriate clinical management and care, as well as for implementing isolation measures to prevent further transmission. False negatives and positives can have significant medical and public health consequences, particularly in the context of COVID-19, where timely and accurate diagnosis is essential for appropriate treatment, isolation, and contact tracing.15,16
A test with high sensitivity is particularly important in identifying individuals who may not exhibit typical symptoms, as well as in detecting early stages of infection before the virus grows to become infectious, or for clinical purposes, to detect remnants of viral infection after an infection is largely cleared in order to link a patient’s recent symptoms to COVID-19. On the other hand, a test with high specificity is crucial for reducing the number of false positives that can lead to unnecessary isolations, quarantining, and resource allocation.
Although high sensitivity and specificity are desirable, there is often a trade-off between these measures and other factors, such as test turnaround time, cost, and ease of administration. Public health officials and clinicians must carefully consider the strengths and limitations of each test in the context of their specific needs and goals, and balance the benefits and risks of testing with other public health interventions.17
Test Sensitivity and Specificity Depend on the Purpose of the Test
Test sensitivity and test specificity usually are considered in the context of medical diagnostic testing, which focuses almost exclusively on the analytic performance of a test to accurately detect the presence or absence of virus RNA or protein when it is present in any amount. A PCR test, for example, with very high analytic sensitivity can theoretically detect down to just a single molecule of the virus.
However, the COVID-19 pandemic demonstrated that there are multiple uses for testing and that what it means to be “positive” or “negative” is not as simple as answering whether or not there is any evidence of the virus in the body.
For COVID-19 testing, the purpose of the test must determine what defines an individual as “positive” or “negative.” Therefore, the purpose of the test impacts how to measure how sensitive or specific the test is.
For instance, during COVID-19, most testing was performed for public health priorities—to identify infectious individuals so that they can isolate and not spread the virus to others. When people are infectious, they usually have millions, billions, or even trillions of copies of virus RNA and protein in their nasal secretions that are collected on the test swabs. If the purpose of a test is to identify people who are currently infectious, then for a test to achieve high sensitivity for identifying infectious people, it does not need to be extremely analytically sensitive and detect down to a single molecule of RNA. Instead, in this example, a test that is highly sensitive to detect currently infectious individuals only needs to detect down to at least a million copies of virus RNA in a swab, and not, for example, 100 copies. This is important because it means that there are many technologies, besides PCR, that can be leveraged that are faster, cheaper, and more accessible than laboratory PCR testing, when the goal is to identify infectious individuals.
A similar line of reasoning exists for specificity of a test as well. With COVID-19, people are generally infectious for approximately 6 days and are usually highly infectious for just 1 to 3 days. However, evidence of the infection in the form of remnant RNA persists for weeks or months after infection. For a test to be specific for identifying people during the infectious period, it should turn negative whenever people are no longer infectious. Therefore, for the purposes of identifying infectious individuals, a test, like PCR, that is extremely sensitive to detect even small remnants of RNA and with great analytic specificity may not be specific at all for the purposes of identifying currently infectious individuals. In fact, because people have remnant RNA in their nose for 3 to 5 times longer than they are infectious, such a test would be most likely to identify people as positive when they are
no longer infectious. Thus, although such a test is useful for medical diagnostic testing, for example, to link lingering symptoms back to an initial COVID-19 infection, a positive laboratory PCR test is more likely to tell someone that they are positive when they are no longer infectious, making it an inappropriate test for identifying individuals who require isolation.
no longer infectious. Thus, although such a test is useful for medical diagnostic testing, for example, to link lingering symptoms back to an initial COVID-19 infection, a positive laboratory PCR test is more likely to tell someone that they are positive when they are no longer infectious, making it an inappropriate test for identifying individuals who require isolation.
Therefore, to properly assess the sensitivity and specificity of a test, it is critical to understand the intended use of the test. A failure to do so, particularly during a pandemic, can result in large amounts of resources being deployed for tests that fail to accomplish the task at hand, while neglecting deployment of much more appropriate tests.2,3
Ethical Considerations of Testing in a Pandemic
Ethical considerations of testing during a pandemic play a crucial role in guiding public health policy and clinical practice. As testing is vital for understanding and controlling the spread of the disease, it is essential to balance individual rights and social justice with public health needs. This subsection will discuss the ethical implications of testing, including privacy versus public health needs, isolating positive individuals, and quarantining contacts of positive individuals.18
Privacy Versus Public Health Needs
The tension between individual privacy and the broader public health needs is a significant ethical challenge in pandemic testing. Although it is crucial to protect personal information, public health authorities may need to access and share sensitive data to track and control the spread of the disease effectively. Balancing these competing interests requires a careful consideration of factors such as the potential harm caused by breaches of privacy, the benefits of sharing information for public health purposes, and the implementation of safeguards to minimize privacy risks. Informed consent, anonymization of data, and strict data-sharing protocols can help address these concerns while still allowing for effective public health interventions.
Isolating Positive Individuals
Isolating individuals who test positive for a contagious disease is an essential public health measure to prevent further transmission. However, this practice raises ethical concerns, particularly around individual autonomy and the potential for social isolation, stigma, and discrimination. To ensure that isolation measures are ethically sound, public health authorities must provide clear and evidence-based guidance on the duration and conditions of isolation, ensuring that individuals have access to appropriate care, support, and information during this period. Additionally, efforts must be made to minimize the potential harms of isolation, such as providing mental health support and addressing the needs of vulnerable individuals who may be disproportionately affected by isolation measures.
Quarantining Contacts of Positive Individuals
Quarantining contacts of positive individuals is another essential public health strategy to control the spread of infectious diseases. This practice involves restricting the movement and social interactions of individuals who have been exposed to the virus but have not yet tested positive or show symptoms. Quarantining raises ethical concerns similar to those surrounding isolation, including the potential infringement on individual autonomy, the risk of social isolation and stigma, and the potential for disproportionate impacts on vulnerable populations.
To address these concerns, public health authorities should ensure that quarantine measures are based on the best available evidence, are time-limited, and are proportionate to the risks posed by the disease. Clear communication regarding the rationale for quarantine, the expected duration, and the support available to those affected is also essential for maintaining public trust and compliance. Additionally, efforts should be made to mitigate the potential harms of quarantine, such as providing financial assistance to those unable to work during this period and ensuring access to essential services and mental health support.
In conclusion, ethical considerations surrounding testing during a pandemic are crucial to ensure that public health interventions are fair, proportionate, and respectful of individual rights. Balancing privacy concerns with public health needs as well as addressing the potential harms associated with isolation and quarantine measures are key aspects of pandemic testing and management.
COVID-19 TESTING ENVIRONMENTS AND OVERVIEW OF THE TESTING TOOLS
The COVID-19 pandemic has led to the development of various testing modalities to accurately diagnose, limit, and monitor the virus’s spread. Although there are many approaches to categorize testing technologies, one major approach is based on the location or environment where the testing itself is performed. Tests can be grouped based on the location where they will be performed: laboratory tests, point-of-care tests, and self-tests. The purpose of the test will generally help define the location and environment in which the test is used and will help define the desired set of test characteristics such as sensitivity, specificity, turnaround time, frequency, cost, and scale. Each of these characteristics must be considered when choosing what type of test to perform while testing for COVID-19. This section will provide an overview of the different types of COVID-19 tests and the types of test environments. In “Molecular Mechanisms and Technologies for COVID-19 Testing” section of this chapter, we will discuss each technology in greater detail.
Testing Environment
Laboratory Testing
Laboratory tests are performed in specialized facilities and typically involve more complex procedures, equipment, and skilled personnel than point-of-care or self-tests. Essentially any test technology can be performed in a laboratory. Examples of laboratory tests for COVID-19 include molecular test or NAATs (eg, PCR, LAMP, Clustered Regularly Interspaced Short Palindromic Repeats [CRISPR]), antigen tests, and antibody tests. When performed in laboratory settings, the tests generally have high sensitivity and specificity, making them particularly valuable for medical use where the tolerable margin of error is very low and the time to get test results back can be days and not minutes.
Advantages of laboratory tests include their high accuracy, the ability to process large numbers of samples, and the potential for adding on tests to specimens, for example, adding sequencing on to positive PCR tests to detect emerging variants. However, laboratory tests can be resource-intensive, require significant logistics to transport specimens to the laboratory and return results to the individual, have longer turnaround times than point-of-care or self-tests, and may be less accessible in remote- or resource-limited settings.
Point-of-Care Testing
Point-of-care tests are designed to be performed at or near the site of patient care, such as a doctor’s office, pharmacy, or community testing site. These tests generally have a faster turnaround time than laboratory tests, with many providing results in as little as 15 minutes or less. Examples of point-of-care tests for COVID-19 include lateral flow antigen tests, rapid molecular NAATs, and lateral flow antibody tests, among others.
Advantages of point-of-care tests include their rapid results, ease of use, and increased accessibility, particularly in settings where laboratory testing may not be readily available. However, point-of-care tests may have lower analytic sensitivity and specificity than laboratory tests, which can, but does not always, result in a higher rate of false negatives or positives. Additionally, these tests may be more susceptible to variations in testing quality because of differences in operator skill and variable adherence to testing protocols.
Self-Testing or Home Testing
Self-tests, also known as at-home or over-the-counter tests, are tests that individuals perform without the need for a healthcare professional. These tests typically involve simple collection and performance procedures, such as nasal swab or saliva collections, and simple-to-use cartridges that you place drops of solution onto or insert swabs into, and can provide results in under an hour, and often within minutes. Self-tests for COVID-19 are often similar or identical to point-of-care tests and include rapid lateral flow antigen tests, rapid NAAT or molecular tests, and lateral flow rapid antibody tests.
Self-tests are advantageous for their convenience, increased accessibility, and potential to reduce the burden on healthcare systems by allowing individuals to test themselves at home. They can also facilitate more frequent testing, which may be particularly valuable for monitoring asymptomatic
or presymptomatic individuals in real time and limiting transmission. However, self-tests may have lower sensitivity and specificity than laboratory or point-of-care tests, and there is a potential for user error or misinterpretation of results.
or presymptomatic individuals in real time and limiting transmission. However, self-tests may have lower sensitivity and specificity than laboratory or point-of-care tests, and there is a potential for user error or misinterpretation of results.
Characteristics of testing, such as sensitivity, specificity, turnaround time, frequency, cost, and manufacturing scale, are crucial factors to consider when selecting the appropriate testing modality for a given situation. Molecular tests performed in a laboratory, such as NAATs, are typically the most sensitive tests, making them ideal for diagnosing infections in a hospital setting where a laboratory may be near to a patient. Point-of-care or self-tests can provide rapid results and are suitable for resource-limited settings or for public health testing where immediate results are critical in order to limit spread.2
The choice of testing modality depends on the specific needs and context of the situation, with each modality offering unique advantages and disadvantages.
Overview of Testing Technologies
Nucleic Acid Amplification Tests
NAATs are a category of molecular tests that detect the presence of specific viral genetic material, making them highly sensitive and specific for diagnosing active infections. NAATs are widely used for COVID-19 testing because of their accuracy and reliability. The most common NAAT technologies used for COVID-19 testing include PCR, LAMP, and transcription-mediated amplification (TMA). New technologies such as CRISPR-based methods are increasing but still have not been as widely used as anticipated for diagnostic assays.19
PCR is the gold standard for COVID-19 testing because of its high sensitivity and specificity. It involves amplifying the virus’s RNA through a series of temperature-controlled reactions, producing a detectable signal if the target genetic material is present. Real-time RT-PCR is a widely used variant that combines RT and PCR in a single process, allowing for the simultaneous amplification and detection of viral RNA. RT-PCR is known for its excellent analytic performance characteristics, but it requires specialized equipment, usually in a high-complexity laboratory, and trained personnel, which may limit its use in some settings.19
LAMP is an isothermal nucleic acid amplification technique that enables rapid detection of viral RNA without the need for complex thermocycling equipment. Because they are isothermal, LAMP assays can be performed at a single, constant temperature, making them more accessible and amenable to point-of-care and self-testing. Although LAMP offers a faster turnaround time than PCR, as a technology it can be, but is not always, slightly less sensitive and less specific than PCR, which could result in higher rates of false-negative or -positive results.19, 20 and 21
TMA is another type of molecular testing that is highly sensitive and specific, similar to PCR and LAMP testing. Like LAMP, TMA testing uses an isothermal reaction to amplify the genetic material of the virus, making it a rapid, reliable, and sometimes more economically viable option for COVID-19 testing.19
CRISPR-based methods for COVID-19 testing leverage the power of CRISPR-Cas systems for target-specific nucleic acid detection. These methods, such as SHERLOCK (Specific High-sensitivity Enzymatic Reporter unLOCKing) and DETECTR (DNA Endonuclease-Targeted CRISPR Trans Reporter), combine isothermal amplification with CRISPR-Cas-mediated detection, resulting in highly sensitive and specific tests. CRISPR-based tests offer rapid turnaround times and can be adapted to point-of-care or high-throughput formats, making them a promising alternative to traditional PCR-based methods.22
Advantages and Disadvantages of NAATs
Advantages of NAATs for medicine and public health include their high sensitivity and specificity, making them highly reliable for diagnosing active infections. They also allow for accurate detection of emerging variants, which is crucial for tracking the spread of new strains and informing public health interventions. However, the disadvantages of NAATs include their reliance on specialized equipment and skilled personnel, which can be resource-intensive and limit their accessibility in remote or resource-limited settings. Additionally, the turnaround time for some NAATs, particularly PCR, can be very long compared to point-of-care or self-tests, reducing or entirely removing their use for identifying currently infectious individuals in time to inform isolation procedures.
Antigen Testing
Antigen tests detect specific viral proteins, called antigens, such as the spike or nucleocapsid proteins of SARS-CoV-2, rather than the virus’s genetic RNA material. These tests are generally faster and simpler to perform than molecular tests, making them suitable for point-of-care or self-testing applications. However, they generally do not include an amplification step, making them less able to detect extremely minute amounts of virus. Examples of antigen tests for COVID-19 include lateral flow assays (LFAs) and enzyme-linked immunosorbent assays (ELISAs).
Lateral flow assays are rapid, portable tests that can provide results within minutes. They work by capturing viral antigens present in a sample, such as a nasal swab, using immobilized antivirus antibodies manufactured as a stripe on an inexpensive nitrocellulose-based test strip that looks much like a piece of paper. If the target antigen is present, it will be captured by the antibodies striped onto the piece of paper and a visible line or signal will appear on the strip. LFAs are widely used for COVID-19 testing because of their simplicity, massive and inexpensive production capacity, rapid turnaround time, and suitability for point-of-care or self-testing.23
Enzyme-linked immunosorbent assays are another type of antigen test that can be used to detect the presence of viral proteins in a sample. Although ELISA-based assays are most commonly known for their detection of antibodies in samples, ELISAs are also often used to detect the virus itself, in a swab or other sample. ELISA-based antigen assays rely on the specific binding of antibodies in the test itself, to viral antigens in the sample of interest, followed by a colorimetric or chemiluminescent detection system to generate a quantifiable signal. These technologies can include extremely sensitive technologies that reach single-molecule detection. Although ELISAs are typically more sensitive than LFAs, they require specialized equipment and trained personnel, making them more suited for laboratory settings.24
Advantages and Disadvantages of Antigen Tests
Advantages of antigen tests for medicine and public health include their rapid turnaround time, ease of use, and suitability for point-of-care or self-testing applications. These characteristics make them valuable for mass testing and frequent screening of asymptomatic individuals, which can help identify and isolate cases more quickly, reducing transmission rates. However, antigen tests generally have lower sensitivity and specificity than molecular tests, particularly in asymptomatic or early-stage infections, which can result in a higher rate of false negatives or positives. Additionally, antigen tests are more difficult to continuously monitor for performance against emerging variants, as compared to NAATs, which can limit their utility for monitoring the spread of new strains because antigen tests may need to be empirically confirmed to perform well whenever a new variant has a mutation in the protein where the antigen test detects.
Antibody Testing
Antibody testing, also known as serologic testing, detects the presence of specific antibodies produced by the immune system in response to a viral infection. These tests are valuable for assessing past infections and immune responses for clinical, public health, and research use, but are not particularly useful for diagnosing active infections because antibodies usually do not form and become detectable until the infection is beginning to or has already been cleared. Examples of antibody tests for COVID-19 include ELISAs, rapid antibody tests, bead-based assays, phage immunoprecipitation sequencing (PhIP-Seq), and array-based antibody testing, among others.
ELISA laboratory tests are widely used for antibody testing because of their high sensitivity and specificity. They involve the specific binding of antibodies in a sample to immobilized viral antigens, followed by a secondary detection system that generates a quantifiable signal. ELISAs can be adapted to detect different classes of antibodies (eg, immunoglobulin M [IgM], immunoglobulin G [IgG], immunoglobulin A [IgA]) and can provide information on the magnitude and duration of the immune response.25
Rapid lateral flow assay antibody tests are point-of-care tests that can detect the presence of specific antibodies within minutes. They use lateral flow technology, similar to rapid antigen tests, but are striped with virus proteins that capture and detect anti-SARS-CoV-2 antibodies in the sample under investigation, rather than the virus itself. Lateral flow antibody tests are designed for ease of use and rapid results. Although rapid antibody tests are more accessible than laboratory-based ELISAs, they may have lower sensitivity and specificity, which can result in a higher rate of false negatives or positives.26
Multiplexed and High-Throughput Methods for Antibody Detection
Advances in technology have led to an explosion of new approaches to measure many antibodies at once, a process called multiplexing. Multiplexing technologies allow for the measurement of the serologic antibody repertoire within samples. Multiplexed testing that can evaluate many analytes simultaneously or technologies that can process many samples at a time are referred to as high-throughput assays and are increasingly useful for large-scale public health programs, such as serologic surveillance, which can be critical to monitor the spread of a virus across whole populations.
Bead-based antibody tests use microscopic beads coated with viral antigens to capture specific antibodies from a sample. A serum sample is applied to the assay and if there are antibodies in the sample that recognize the viral proteins coated on the beads, those particular beads will be detectable by an instrument. The most common of these is a technology called Luminex beads. These assays offer multiplexing capabilities, allowing for the simultaneous detection of antibodies against multiple viral proteins or variants. Bead-based assays can provide valuable information on the breadth and specificity of the immune response but require highly specialized equipment and trained personnel.27
Phage immunoprecipitation sequencing or PhIP-Seq antibody testing is a high-throughput serology method that combines a technology called phage display with NGS to profile the antibody repertoire of an individual. This technique can provide a detailed understanding of the immune response to SARS-CoV-2 and inform vaccine development and evaluation efforts. PhIP-Seq is a new technology and exists primarily within academic research laboratories, but is increasingly being made available for public health testing.28
Array-based antibody testing involves immobilizing viral antigens on a solid support, such as a glass slide or silicon chip, and probing the array with a sample to detect the presence of specific antibodies. This technology enables high-throughput, multiplexed analysis of antibody responses and can be used to monitor immune responses to different viral strains or vaccine formulations.28,29
Advantages and Disadvantages of Antibody Tests
Antibody testing for medicine and public health offers the ability to assess past infections and immune responses, which can inform epidemiologic studies, vaccine development, and immunity-based surveillance efforts. However, antibody testing is limited in its utility for diagnosing active infections, and the potential for cross-reactivity with antibodies against other coronaviruses can complicate estimates of infection prevalence.
Sequencing
The rapid emergence of new SARS-CoV-2 variants throughout the pandemic has necessitated unprecedented rapid and widespread implementation of genomic sequencing techniques to track virus transmission, evolution, and emergence of novel variants. Sequencing the SARS-CoV-2 genome is invaluable for monitoring and tracking the virus’s behavior to inform public health strategies aimed at controlling the spread of the disease and monitor emergence of variants of concern, for instance, those that may evade immunity acquired through vaccination or previous infection.
There are several sequencing technologies available, each with its unique characteristics and applications. The three main types of sequencing technologies are short-read sequencing, long-read sequencing, and nanopore sequencing.
Short-read sequencing, such as Illumina’s sequencing-by-synthesis technology, generates millions of relatively short DNA fragments (usually up to 300-600 base pairs). This high-throughput approach allows for the rapid generation of large amounts of genomic data at a relatively low cost per base. However, short-read sequencing can struggle with resolving complex genomic regions, such as repetitive sequences or structural variations.30
Long-read sequencing, exemplified by Pacific Biosciences’ (PacBio) single-molecule real-time (SMRT) sequencing, and Oxford Nanopore’s nanopore sequencing generate much longer reads (up to tens of thousands of base pairs) than short-read sequencing. The benefit of this technology over short-read sequencing is that it can provide a more complete view of complex genomes and help resolve structural variations. However, long-read sequencing typically has a higher error rate (though coming down quickly) and can be more expensive than short-read sequencing.31
The choice of sequencing platform depends on the specific research question and resources available. Illumina’s short-read sequencing is known for its high accuracy, scalability, and cost-effectiveness, making it suitable for large-scale genomic studies and routine surveillance efforts. PacBio’s long-read sequencing offers improved resolution of complex genomic regions and structural variations, which
can be important for understanding the functional consequences of viral mutations. Nanopore’s long-read, real-time sequencing using rapid and portable solutions is ideal for viral surveillance, outbreak investigations, and field-based research where time, access, and affordability are critical.
can be important for understanding the functional consequences of viral mutations. Nanopore’s long-read, real-time sequencing using rapid and portable solutions is ideal for viral surveillance, outbreak investigations, and field-based research where time, access, and affordability are critical.
Advantages and Disadvantages of Sequencing
Sequencing for medicine and public health offers the ability to track and monitor viral variants, inform diagnostic and therapeutic development, and offer insights into viral transmission and evolution. By leveraging these strengths, sequencing technologies can contribute to a comprehensive understanding of the virus and help guide public health interventions.
However, sequencing is not suited for certain applications in COVID-19 testing. Although it is highly accurate and provides deep insights into the virus being detected, it is slower and much more costly than PCR or rapid antigen testing. Although it can be useful for large-scale surveillance, exemplified by its use in sequencing of wastewater for identification of SARS-CoV-2 genetic material, it is less useful for diagnostic use or public health screening and real-time surveillance for limiting outbreaks.
Sample Types and Specimen Collection
A test is only as good as the sample that is provided to it, and sample types play a crucial role in determining the accuracy and effectiveness of COVID-19 testing. Selecting the appropriate sample type for the use case (ie, medical diagnostics, public health screening, population surveillance) is critical and influences test sensitivity, ease of collection, patient comfort, and overall ability to detect the virus or, in the case of immunity-based testing, evidence of past infection or immunity to the virus. This section describes the major sample types used for testing during the COVID-19 pandemic, including nasopharyngeal swabs, oropharyngeal swabs, nasal swabs, saliva samples, blood collections, and self-collected samples.
Testing for the Virus
The material collected, for example, on a swab or in saliva, is the only biologic link between the test itself and whether or not the virus is detected for the individual. So the choice of specimen collection is critical and must carefully take into consideration the advantages and disadvantages of each collection or specimen type.
Nasopharyngeal Swabs
Nasopharyngeal swabs involve collecting samples from the back of the nasal cavity called the nasopharynx. This method is often considered the gold standard for testing for respiratory viruses, including COVID-19. Many respiratory viruses, including SARS-CoV-2, tend to grow well in the nasopharynx and thus a swab that collects a specimen from the nasopharynx has a high sensitivity to pick up the virus. However, the procedure can be uncomfortable for patients, often causing gagging or sneezing, and requires trained personnel to perform the swabbing correctly, ensuring a proper sample is collected.
Nasopharyngeal swabs are well-suited for both NAAT and antigen tests. They are ideal for hospital and clinical settings, where the highest sensitivity specimen collection is required for diagnosis and patient management and where healthcare professionals are present to perform the swabbing. During the early stages of the pandemic, nasopharyngeal swabs were the predominant specimen type used for COVID-19 testing. However, as the pandemic progressed, the extra burden on both the test recipient and the resources required to perform the nasopharyngeal swab caused these types of swabs to largely fall out of favor except in hospitals and doctors’ offices.
Nasal Swabs
Nasal swabs involve collecting samples from the lower part of the nasal cavity or the nares. Nasal swabs have been considered less sensitive than a nasopharyngeal swab because the virus niche is more closely aligned with the nasopharynx than the nares, and thus a nasal swab may be slightly less likely to pick up virus in the nares. However, the much greater ease with which a nasal swab can be performed, including a self-swab, and the relative comfort of a nasal swab have caused nasal swabs to become a primary mode of sample collection. This is especially true for self-sampling for home testing where nasopharyngeal swabs are not practical.
Nasal swabs, like nasopharyngeal swabs, are suitable for both NAAT and antigen testing. They are commonly used in mass testing or screening programs, where ease of collection and patient comfort are prioritized and healthcare professional resources are limited.
Oropharyngeal Swabs
Oropharyngeal swabs involve collecting samples from the back of the throat. Similar to nasopharyngeal swabs, oropharyngeal swabs may cause discomfort for patients and often require trained healthcare professionals to collect the samples correctly. During COVID-19 however, many parts of the world, particularly throughout Europe, encouraged oropharyngeal swabs for self-tests, either alone or in combination with self-collected nasal swabs, demonstrating that these types of swabs and specimens can be reliably performed at home by non-healthcare professionals, including people who are self-swabbing.32
For COVID-19 testing, oropharyngeal swabs have slightly lower sensitivity over the duration of the infection. However, low-grade evidence suggests that the virus may be more readily detectable in the oropharynx very early in the infection.33 Therefore, oropharyngeal swabs are usually used in combination with nasal or nasopharyngeal swabs. By sampling more than a single site, the performance of the combined swabs tends to be better than any single site alone. Despite data demonstrating that the addition of an oropharyngeal swab to a nasal swab improves overall sensitivity to detect the virus in an infected individual,32 and oropharyngeal swabs being deployed safely across Europe, in the United States, the FDA has discouraged the use of oropharyngeal swabs, warning that these swab types may pose too much of a safety hazard for home testing. This reflects a pattern of a much more conservative stance by the FDA in terms of bringing needed tools to individuals during a public health emergency, compared to many other nations.34
Like nasopharyngeal and nasal swabs, oropharyngeal swabs are suitable for both NAAT and antigen-based testing.
Saliva Collection
Saliva samples are noninvasive and can be easily collected by the patients themselves. They offer a high level of patient comfort and convenience and are thus powerful specimen collection types for large-scale public health screening and surveillance. Saliva samples are commonly considered to have lower sensitivity overall, across the full course of an infection, compared to nasopharyngeal and nasal swabs, in part because the viral load in saliva can vary significantly across individuals. On the other hand, for COVID-19 testing, limited evidence suggests that saliva specimens may be superior to nasopharyngeal and nasal specimens during the initial 2 days of infection. Scientists hypothesize that the virus growth patterns and early kinetics may cause the virus to be abundant initially in the saliva before replicating to high enough levels in the nasopharynx or nares to be detectable.33
Saliva samples are generally most suitable for NAAT, including PCR and LAMP assays, as well as some laboratory-based antigen tests. Although nasopharyngeal, nasal, and oropharyngeal swabs are often used for specimen collection for rapid antigen testing, saliva-based samples are generally not used for rapid antigen tests. As of this writing, there is one rapid NAAT authorized by the FDA for home use that is meant to be used with a saliva specimen.35
The ease of saliva sample collection makes saliva specimens appropriate for use in large-scale public health screening programs where ease of collection, low-resource requirements, and patient comfort are prioritized. For example, the FDA authorized the first saliva-based COVID-19 laboratory PCR test developed by Rutgers University in April 2020, which was later used for widespread testing in various settings. Saliva-based collections also form the basis of the widely used SalivaDirect laboratory PCR test that benefited many school, university, and workplace testing programs in large part because of its simple saliva-based specimen collection. Of note, the SalivaDirect test also was an advancement because it replaced a major bottleneck in laboratory testing procedures, RNA extraction, with simple heating of the saliva specimen, making the laboratory test more resource friendly, particularly in the beginning of the pandemic when RNA extraction kits became the limiting resources for lab-based testing.36
Exhaled Breath—An Emerging Specimen Type
Exhaled breath is a promising albeit still unproven specimen and sample collection approach for COVID-19 testing. Exhaled breath collection involves, as the name suggests, collecting the exhaled breath from individuals. Methods and technologies for exhaled breath–based collection are currently under investigation.37
In general, exhaled breath collection involves using a specialized device to collect and concentrate the exhaled breath. These devices can be portable or stationary, and they work by directing the exhaled breath through a filter or membrane that traps the aerosolized particles, including the virus. Once collected, the sample is then eluted off of the filter or membrane, usually by a test buffer or saline solution, and the eluted specimen in the buffer is analyzed for the presence of SARS-CoV-2
RNA or antigen using standard PCR or antigen tests, as well as other less conventional assays such as electrochemical or mass spectrometry–based tests.36,38
RNA or antigen using standard PCR or antigen tests, as well as other less conventional assays such as electrochemical or mass spectrometry–based tests.36,38
Compared to other specimen collection methods such as swabs or saliva, breath-based collection has several potential advantages. It is noninvasive and ideally would not require the use of invasive techniques or specialized training for sample collection. Compared to a swab, breath-based collection has the potential to be more comfortable for patients than swabs, requiring only breathing out a number of times onto a collection device. Improved comfort of a noninvasive test might reduce anxiety associated with sample collection, which can be particularly useful for public health screening tests. Additionally, because of their novelty, breath-based collections use distinct supply chains compared to nasal swabs and could provide public health testing programs an alternative to traditional collection methods when swabs are in short supply, such as happened during the first months of the COVID-19 pandemic.
However, there are several challenges associated with breath-based collection for COVID-19. One key challenge is the variability in the concentration and amount of virus particles in exhaled breath, which can affect the sensitivity and accuracy of test results. Specifically, the kinetics of when virus particles exist in the exhaled breath as compared to when they can be detected on a nasal swab may be distinct and this could cause confusion when comparing the two methods. For example, early evidence suggests that virus load may be detectable in the breath earlier in the course of an infection compared to detection via nasal swab. However, the viral load may then drop out of the exhaled breath faster as the patient begins to clear the infection, compared to nasal swab. In such a situation, the sensitivity of exhaled breath as a collection modality may be higher at the beginning of an infection and lower toward the end of an infection, as compared to a nasal swab.39 Significantly greater amounts of data are needed to make exhaled breath viable as a specimen type to be trusted and interpreted for clinical and public health purposes. Additionally, the collection efficiency may vary depending on factors such as the patient’s age, sex, and underlying health conditions, which can further impact the accuracy of the test results. As a relatively new approach, the potential for exhaled breath–based collection exists, but more research is needed to determine the utility and effectiveness of breath-based collection for COVID-19 testing in practice.
Self-Collected Versus Healthcare Professional–Collected Specimens
Self-collected samples, such as nasal swabs, oropharyngeal swabs, and saliva samples, offer several advantages for testing during a public health emergency, including increased patient comfort, reduced burden on healthcare professionals, and the potential for substantially increasing access to testing, particularly through at-home testing. However, self-collection can be difficult at first and may result in lower-quality samples or incorrect collection techniques for those not yet acquainted with self-testing, which could affect the tests’ performance.40
Importantly, most data demonstrating reduced performance of self-collected samples as compared to healthcare professional–collected samples looked only at the initial attempt for an individual collecting their own sample. As individuals performed self-collections second, third, and fourth times however, the difference between self-collection and professional collection reduces toward no difference. Additionally, by now, many or most humans have performed a self-swab and thus the early data suggesting reduced accuracy with self-swabs may no longer be relevant. This reflects the challenges with how quickly data can become obsolete and, more generally, how well data are able to keep up with the changing nature of a pandemic such as COVID-19.41,42
Self-collected samples are appropriate for large-scale testing programs. The lack of professional resource requirements makes them especially useful when rapid and frequent testing is required to monitor the spread of the virus in a community.40,42
For public health programs, the ideal sample type should balance sensitivity, ease of collection, and patient comfort. Nasal swabs and saliva samples are particularly well-suited for these purposes because of their noninvasive nature, ease of collection, and suitability for self-collection. They are appropriate for large-scale testing, screening, and surveillance efforts.
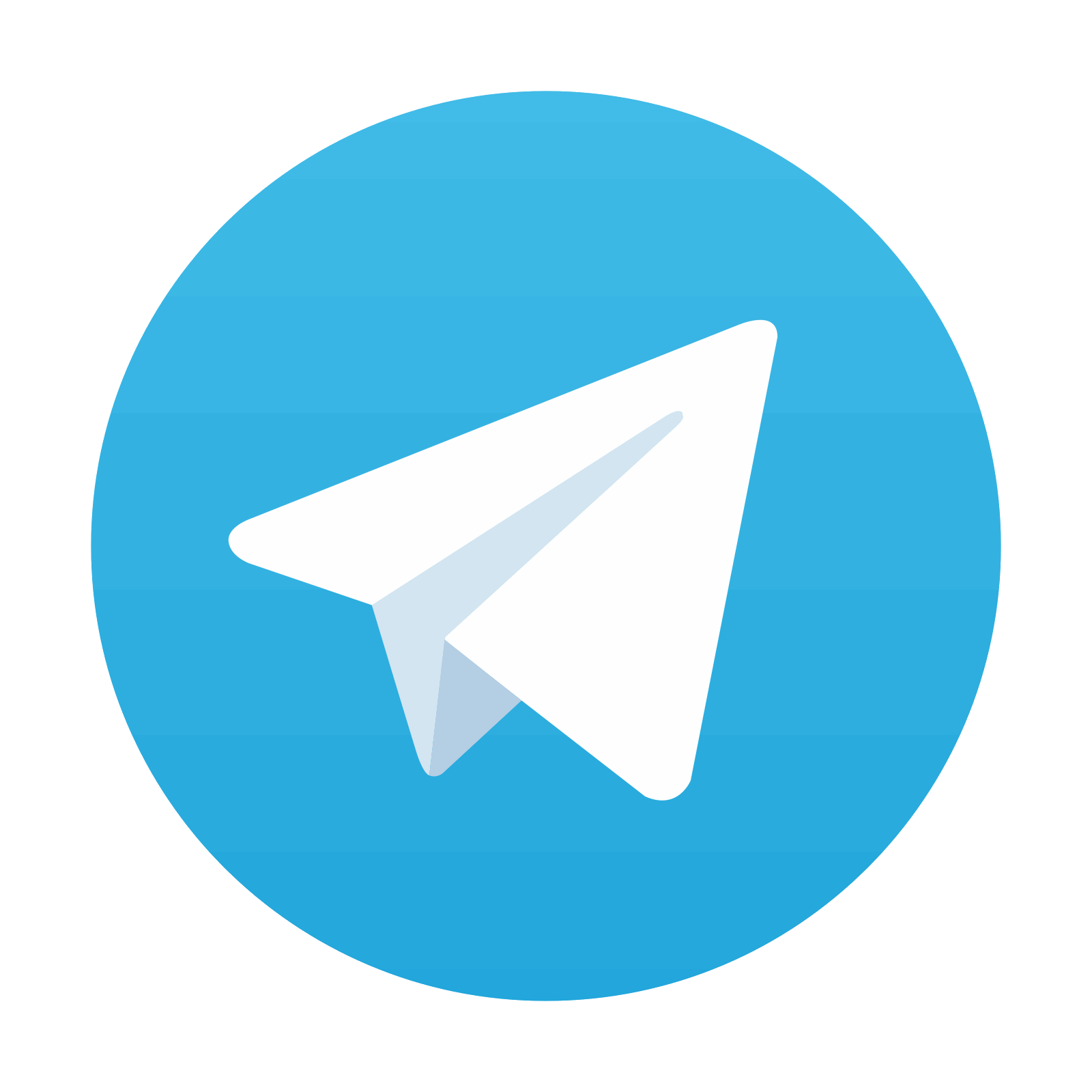
Stay updated, free articles. Join our Telegram channel

Full access? Get Clinical Tree
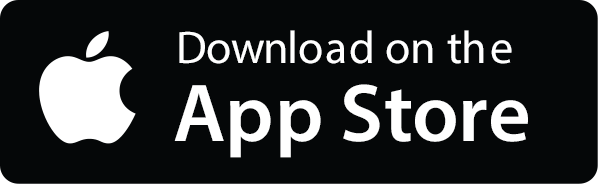
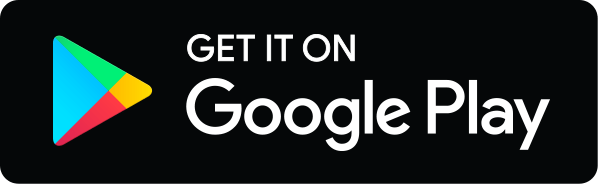
