Abstract
Infertility is a major public health concern affecting up to 25 percent of couples worldwide [1]. It is defined by the failure of a couple to achieve conception after one year of regular, unprotected intercourse. The male contributes to roughly 50 percent of the causes of infertility among couples highlighting the importance of research and development in this field of medicine [2]. Conventional semen analysis is the cornerstone test for evaluating the male factor [3]. While the test can provide a general understanding of the male fertility potential, it cannot be used as an accurate predictor of fecundity. About 10 percent of men with normal semen analysis are unable to conceive. On the contrary, an equal percentage of men with abnormal semen analysis have no issues with fertility [4]. Therefore, during the past few decades, the low predictive power of standard semen analysis has triggered researchers to look for more sensitive and specific tests of sperm function. This chapter aims to explore the clinical utility of various tests of sperm function that have been described in literature.
24.1 Introduction
Infertility is a major public health concern affecting up to 25 percent of couples worldwide [1]. It is defined by the failure of a couple to achieve conception after one year of regular, unprotected intercourse. The male contributes to roughly 50 percent of the causes of infertility among couples highlighting the importance of research and development in this field of medicine [2]. Conventional semen analysis is the cornerstone test for evaluating the male factor [3]. While the test can provide a general understanding of the male fertility potential, it cannot be used as an accurate predictor of fecundity. About 10 percent of men with normal semen analysis are unable to conceive. On the contrary, an equal percentage of men with abnormal semen analysis have no issues with fertility [4]. Therefore, during the past few decades, the low predictive power of standard semen analysis has triggered researchers to look for more sensitive and specific tests of sperm function. This chapter aims to explore the clinical utility of various tests of sperm function that have been described in literature.
24.2 Sperm Function Tests in the Clinical Setting
24.2.1 Measures of Oxidative Stress
24.2.1.1 Background on the Implications of Oxidative Stress on Male Fertility
Oxidative stress (OS) is believed to be an important common mechanism, through which various etiologies of male factor infertility may cause sperm dysfunction. It results from the imbalance between oxidants and reductants in any given medium. Oxidants, also known as reactive oxygen species (ROS) are the products of aerobic metabolism that, under normal physiologic levels, play an important role in cell signaling [5]. However, several disease processes such as varicocele, genitourinary infection or trauma, malignancy and its treatment as well as various environmental exposures can cause an excessive production of ROS [6]. Antioxidants, on the other hand, are compounds that are capable of eliminating excessive ROS, thereby minimizing their unwanted effects. Nonetheless, when ROS production exceeds the antioxidant neutralizing capacity, OS occurs resulting in detrimental effects on sperm function. OS is believed to aggravate sperm lipid peroxidation, DNA fragmentation and abortive apoptosis thereby causing infertility [7, 8].
Due its small amount of cytoplasm with only little antioxidants available that can protect it from excessive ROS, the sperm cell is highly susceptible to OS [9, 10]. Furthermore, its plasma membrane contains large amounts of polyunsaturated fatty acids that are extraordinarily prone to peroxidation by ROS [11, 12]. High levels of ROS were detected in semen samples from up to 40 percent of men with infertility [5].
Studies have revealed a significant negative impact of OS on various semen parameters. Homa et al. [13] compared the ROS levels between three groups of patients; normal semen without leukocytospermia, abnormal semen without leukocytospermia and any semen with leukocytospermia. The authors reported the highest ROS levels in patients with leukocytospermia. More importantly, in patients without leukocytospermia, significantly higher levels of ROS were identified in men with abnormal semen than those with normal semen parameters. Similarly, Agarwal et al. [14] compared semen parameters and ROS levels between infertile patients and normal fertile controls. The authors confirmed the presence of higher ROS levels in semen samples of infertile patients in comparison to the fertile controls and reported significant positive associations between ROS levels and sperm concentration and total motility.
Excessive ROS can also impact sperm morphology. Venkatesh et al. [15] examined ROS between infertile patients and fertile men. The median ROS level was 124 times higher in infertile patients who had a significantly higher percentage of sperm with cytoplasmic droplets indicating that it might be secondary to OS. Recently, we have examined OS measures in semen samples obtained from 1168 infertile patients and 100 fertile men and detected a significantly negative correlation between OS measures and sperm normal morphology and a significantly positive correlation between OS measures and percentage of head defects [16].
Finally, several studies have confirmed the relationship between OS and sperm DNA fragmentation (SDF) [7, 17, 18, 19, 20]. SDF is an important cause of male factor infertility and will be discussed later in this chapter.
24.2.1.2 Tests Used for the Measurement of Oxidative Stress
Oxidative stress testing has gained much interest in recent years, especially after understanding its negative effects on male factor infertility. Various tests for OS measurement are available and can be roughly classified into direct and indirect methods. Direct methods assess the extent of oxidation within a spermatozoon, while indirect methods measure the detrimental consequences of OS such as lipid peroxidation or SDF. Table 24.1 lists the various methods for OS measurement. The technical aspects of the different OS measurement methods are discussed in Chapter 7, Sections 7.1 and 7.2.
Table 24.1 Types of Reactive Oxygen Species Measurement Assays
Direct assays | Indirect assays |
---|---|
Chemiluminescence | Total antioxidant capacity |
Flow cytometry | Sperm DNA fragmentation |
Nitroblue tetrazolium test | Chemokines |
Thiobarbituric acid assay | Oxidation reduction potential |
Cytochrome C reduction test | Myeloperoxidase/Endtz test |
Electron spin resonance |
24.2.1.3 Indications and Clinical Value of Oxidative Stress Measurement
Although measuring OS in semen is not indicated during the routine evaluation of male fertility potential, it may provide valuable information in specific clinical scenarios. These include patients with clinical varicocele, lifestyle or environmental risk factors, genitourinary infections or leukocytospermia and couples going for assisted reproductive therapy.
Varicocele is the most common correctable cause of male infertility occurring in 40 percent and 80 percent of men with primary and secondary infertility, respectively [21]. Current evidence suggests that OS plays an integral role in the pathophysiology of sperm dysfunction in varicocele patients [22]. Several studies have confirmed the presence of elevated levels of OS markers in semen samples of men with varicocele compared to normal controls [22, 23, 24, 25]. Allamaneni et al. [26] reported a positive correlation between seminal ROS levels and varicocele grade, meaning that men with larger varicoceles had significantly higher seminal ROS levels than men with small varicoceles. Among healthy fertile men, varicocele appears to be associated with significantly higher levels of ROS in comparison to men without varicocele [27]. Specific free radicals such as NO [25, 28, 29, 30] and H2O2 [31, 32] were found to be significantly higher in semen samples from infertile men with varicocele in comparison to normal fertile controls.
Interestingly, surgical treatment of varicocele has been shown to reduce seminal oxidative stress in varicocele patients [33]. In one study, Sakamoto et al. [25] found that a time lag of approximately six months is required to achieve a marked improvement in seminal ROS markers after varicocele repair. Mostafa et al. [34] demonstrated a significant reduction of markers of seminal OS and an elevation of antioxidant levels three and six months after varicocele ligation. Finally, Hurtado de Catalfo et al. [35] reported normalization of seminal levels of antioxidant enzymes compared with age-matched fertile controls after varicocele ligation. These data indicate that OS measurement could play a vital role in evaluating surgical candidates for varicocele ligation and in following up the response to the treatment.
Oxidative stress measurement may also be indicated in patients with lifestyle/environmental exposures. Smoking is one factor that has been associated with significantly increased seminal ROS levels. Saleh et al. [36] compared infertile smokers and non-smokers with a group of healthy non-smoking fertile men. These authors observed 107 percent higher ROS levels among the smoking group in comparison to the infertile and fertile non-smoker groups. Another study has revealed that even among fertile men, smoking tends to be associated with significant elevations in seminal ROS levels [37]. Patients with certain occupational exposures may also benefit from OS measurement. Examples of such exposures include polyvinyl chloride (PVC) [38], heavy metals such as cadmium and lead [39], and polycyclic aromatic hydrocarbons (PAHs) [40], which have been associated with increased levels of seminal ROS and worse semen quality among exposed patients.
Finally, seminal OS measurement may also be considered in couples opting for ART especially in cases who had prior history of ART failure or recurrent miscarriages following ART. High seminal OS measures can aggravate SDF and alter the outcome of fertilization and embryogenesis [41]. Furthermore, many of the procedures performed during the course of in vitro fertilization or intra-cytoplasmic sperm injection may expose the sperm, oocyte and/or the embryo to exogenous sources of ROS. Examples of these procedures include the degree of illumination [42], culture media being used [43], the incubator temperature [44], centrifugation time [45] and the process of cryopreservation [46]. Therefore, prior identification and treatment of seminal OS in patients undergoing ART could help in ameliorating its negative effects on the ART outcome.
24.2.2 Sperm DNA Fragmentation Testing
24.2.2.1 Background on the Effects of High Sperm DNA Fragmentation on Male Fertility
The study of SDF has certainly gained a lot of attention over the past couple of decades. Sperm DNA integrity is believed to play an important role in fertilization, early embryo development and pregnancy [47, 48]. The human sperm is a unique cell as its DNA is in a highly compacted state aided by the replacement of its histones as nucleoproteins with protamines during spermiogenesis [49]. This compact state provides protection to the DNA as the sperm travels through the male and female reproductive tracts. However, a number of etiologies can trigger SDF such as errors in chromatin compaction during spermiogenesis, elevated seminal ROS levels or aggravated apoptosis during the sperm transit through the epididymis [50, 51]. There is a large body of evidence suggesting a significant relationship between SDF and abnormal semen parameters and worse fertility outcomes both naturally and following ART [49, 52, 53, 54, 55, 56]. A meta-analysis of 28 studies involving 2883 infertile men and 1294 fertile men revealed significantly higher SDF levels among infertile patients in comparison to fertile controls [57].
24.2.2.2 Tests Used in the Measurement of Sperm DNA Fragmentation
There are two types of assays that have been developed to measure SDF: those that can directly measure the extent of DNA fragmentation through the use of probes and dyes and those that measure the susceptibility of DNA to denaturation, which occurs more commonly in fragmented DNA. The eight described methods to assess SDF are briefly presented below and summarized in Table 24.2. The most commonly used tests are terminal deoxynucleotidyl transferase dUTP nick end labeling (TUNEL), the sperm chromatin dispersion test (SCD), and the sperm chromatin structure assay (SCSA).
Table 24.2 Principle, Advantages and Disadvantages of Various Sperm DNA Fragmentation Testing Methods
Test | Principle | Advantage | Disadvantage |
---|---|---|---|
(1) AO test | Metachromatic shift in fluorescence of acridine orange when bound to ss DNA. Uses fluorescent microscopy. | Rapid, simple and inexpensive. | Inter-laboratory variations and lack of reproducibility. |
(2) AB staining | Increased affinity of AB dye to loose chromatin of sperm nucleus. Uses optical microscopy. | Rapid, simple and inexpensive. | Inter-laboratory variations and lack of reproducibility. |
(3) CMA3 staining | CMA3 competitively binds to DNA indirectly visualizing protamine deficient DNA. Uses fluorescent microscopy. | Yields reliable results as it is strongly correlated with other assays. | Inter-observer variability. |
(4) TB staining | Increased affinity of TB to sperm DNA phosphate residues. Uses optical microscopy. | Rapid, simple and inexpensive. | Inter-observer variability. |
(5) TUNEL | Quantifies the enzymatic incorporation of dUTP into DNA breaks. Can be done using both optical microscopy or fluorescent microscopy. Uses optical microscopy, fluorescent microscopy and flow cytometry. | Sensitive, reliable with minimal inter-observer variability. Can be performed on few sperm. | Requires standardization between laboratories. |
(6) SCSA | Measures the susceptibility of sperm DNA to denaturation. The cytometric version of the AO test. Uses flow cytometry. | Reliable estimate of the percentage of DNA-damaged sperm. | Requires the presence of expensive instrumentation (flow cytometer) and highly skilled technicians. |
(7) SCD or Halo test | Assess dispersion of DNA fragments after denaturation. Uses optical or fluorescent microscopy. | Simple test. | Inter-observer variability. |
(8) SCGE or Comet assay | Electrophoretic assessment of DNA fragments of lysed DNA. Uses fluorescent microscopy. | Can be done in very low sperm count. It is sensitive and reproducible. | Requires an experienced observer. Inter-observer variability. |
(1) Acridine orange (AO) stains normal DNA fluoresces green, whereas denatured DNA fluoresces orange-red. (2) Aniline blue (AB) staining showing sperm with fragmented DNA and normal sperm. (3) Chromomycin A3 (CMA3) staining: protamine deficient spermatozoa appear bright yellow, spermatozoa with normal protamine appear yellowish green. (4) Toluidine blue (TB) staining: normal sperm appear light blue and sperm with DNA fragmentation appear violet. (5) Terminal deoxynucleotidyl transferase-mediated fluorescein-deoxyuridine triphosphate-nick end labeling (TUNEL).
24.2.2.3 Indications and Clinical Value of Sperm DNA Fragmentation Testing
The significant impact SDF plays on fertility potential yielded the publication of society endorsed guidelines on the utility of SDF testing in clinical practice [58]. SDF testing is indicated in patients with unexplained infertility, recurrent miscarriages, varicocele, recurrent intrauterine insemination (IUI) and IVF failure, and recurrent miscarriages following ICSI [59].
Unexplained infertility is observed in about 10–30 percent of couples seeking fertility and is perhaps a true demonstration of the limitations of conventional semen analysis [60]. While the SDF value is inversely related to conventional semen parameters, high SDF levels may as well be detected in patients with normal semen analysis result [61]. Oleszczuk et al. confirmed the presence of significantly higher SDF levels in patients with unexplained infertility (18 percent) in comparison to fertile controls (10 percent) (p=0.005) [62]. Similarly, Saleh et al. observed a SDF index of 23 percent (assessed with SCSA) in men with unexplained infertility which was significantly higher than the values measured in fertile controls (15 percent) [19].
A number of studies have linked the SDF level to recurrent early miscarriages, defined by three consecutive pregnancy losses before 20 weeks’ gestation [55, 63, 64]. Khadem et al. measured SDF using the SCD assay in 30 patients with recurrent spontaneous abortions (RSA) and an equal number of fertile men [63]. The authors observed significantly higher SDF levels in patients with RSA (43.3 percent) compared with fertile controls (16.7 percent) (p=0.027). A recent systematic review and meta-analysis by Tan et al. conducted on 12 prospective and two retrospective studies involving 530 men with a history of RSA and 639 fertile controls revealed significantly higher SDF levels in patients with RSA compared with fertile men (mean difference 11.98, p<0.001) [65].
Varicocele is another condition in which SDF testing is indicated. A literature review by Zini and Dohle examined 16 case-control studies and reported that varicocele is associated with higher SDF levels regardless of the patients’ fertility status [66]. A multicenter study by Esteves et al. examining SDF levels among patients with different etiologies of infertility observed the highest SDF levels in patients with varicocele (35.7±18.3 percent) [67]. Similarly, Smith et al. have echoed similar results and identified high SDF values in varicocele patients with normal or abnormal semen parameter results [68].
More importantly, varicocelectomy has been associated with a sound and significant reduction in SDF results [24, 69, 70]. A literature review by Zini and Dohle observed reductions in SDF levels in all the studies included in their study [71]. Another meta-analysis reported a significant reduction in SDF with a mean difference of −3.37 percent (95 percent CI, −4.09 to −2.65; p<0.00001) in patients undergoing varicocelectomy [72]. The reductions in SDF levels following varicocele treatment have been linked with improved pregnancy rates in a study by Smit et al [73]. The authors examined 49 patients who underwent varicocelectomy after a one-year history of infertility. A significant decrease in SDF from 35.2 percent to 30.2 percent (p=0.019) measured by SCSA was observed postoperatively. Natural pregnancy was observed in 37 percent of patients who had a significantly lower SDF than patients who did not conceive naturally or who conceived with assisted reproduction [73].
As for ARTs, a direct relationship has been observed between the SDF level and poor IUI, IVF and ICSI outcomes. Duran et al. evaluated the outcome of 154 IUI cycles and detected significantly higher SDF levels among patients with failed cycles [74]. Similarly, Bungum et al. reported lower pregnancy (3 percent versus 23.7 percent) and delivery (1 percent versus 19 percent) rates among patients with higher versus lower SDF levels [75]. With regards to IVF and ICSI, the current evidence features a significantly negative impact for SDF only on IVF pregnancy rates with reported odds ratios ranging between 1.27 (95 percent CI, 1.05–1.52; p=0.01) [76] and 1.57 (95 percent CI, 1.18–2.07; p<0.05) [77]. Nonetheless, higher SDF levels were associated with increased risk of pregnancy loss following both IVF and ICSI with a combined OR ranging between 2.16 (95 percent CI, 1.54–3.03, p<0.001) [78] and 2.48 (95 percent CI, 1.52–4.04; p<0.0001) [77].
24.2.3 Sperm Penetration Assay
24.2.3.1 Rational for Conducting the Sperm Penetration Assay
The SPA is one of the first tests utilized to assess sperm function. It is an in vitro test that combines sperm subjected to capacitating conditions with hamster eggs that are enzymatically devoid of their zona pellucida. The SPA assesses the sperm ability to perform capacitation and acrosome reaction and to fuse and penetrate through the oolemma and decondensate within the cytoplasm of hamster oocytes [79].
24.2.3.2 Indications and Clinical Value
The SPA has been thoroughly investigated in the pre-ICSI era [80, 81]. However, interest in this time-consuming and expensive test soon faded away principally because of its inconsistent results and the presence of significantly high false-positive and false-negative rates [82]. The reason behind these findings is believed to be related to dissimilarity the SPA has from the normal physiologic process in which the zona pellucida is absent.
A meta-analysis by Oehninger et al. performed to determine the predictive value of various sperm function tests on fertilization outcome with IVF reported a poor clinical value for the SPA [79]. The authors investigated a total of 2906 cycles, and found that although the SPA had a fairly good sensitivity, it suffered from very high false-positive rates averaging about 50 percent. Another meta-analysis by Bol et al. exploring 647 patients from 24 studies reported a SPA sensitivity of only 37 percent and highlighted the heterogenous nature of the reported results [83].
24.2.4 Sperm Zona Pellucida Binding Assays
24.2.4.1 Rational for Conducting the Sperm Zona Pellucida Binding Assay
The interaction between the sperm and the zona pellucida is an important step in the process of conception that can highlight fundamental sperm functional ability; namely capacitation and acrosome reaction. The most commonly used sperm-zona pellucida binding tests are the hemizona assay (HZA) and the competitive intact zona sperm binding test [84, 85]. Both tests are conducted to assess the tight binding of the sperm to the zona pellucida. The HZA uses matching halves of a human zona pellucida from stored or cadaveric oocytes to compare the binding ability of spermatozoa between infertile patients and fertile controls [84]. Studies have shown that sperm from fertile men have significantly higher binding capacity to the zona pellucida than sperm from infertile men [84, 86]. Results of the HZA are expressed as the hemizona index (HZI) which is calculated as follows: bound sperm from infertile male divided by bound sperm from fertile male × 100. The competitive intact zona sperm binding test, on the other hand, uses oocytes that failed to fertilize in vitro to compare control and test spermatozoa [87]. Both of these tests have been shown to demonstrate a high power in predicting fertilization in vitro [88, 89].
24.2.4.2 Indications and Clinical Value
Sperm zona pellucida binding assays are indicated in patients with recurrent fertilization failure during IVF or in the presence of moderate to severe derangement of semen parameters. A meta-analysis investigating 978 couples reported a significantly high predictive power for sperm zona pellucida binding assays for fertilization outcome with a positive predictive value >80 percent and a negative predictive value >70 percent [79]. Studies have reported a HZI cut-off of 35 percent to be a predictive value for IVF outcome [90–92]. Furthermore, a HZI <30 percent was associated with a significantly lower pregnancy rate following IUI in comparison to patients with a HZI >30 percent (11.1 percent versus 40.6 percent, respectively; p<0.05) [93]. Sperm from oligozoospermic men have significantly lower sperm – zona pellucida binding capacity consistent with their lower fertility potential both naturally and following ARTs [94]. Therefore, the result of these sperm function tests could help in counseling patients with moderate to severely abnormal semen parameters before deciding the most appropriate ART method for them. ICSI would be more reasonable for patients with abnormal sperm zona pellucida binding test results [95, 96].
24.2.5 Acrosome Reaction
24.2.5.1 Rational for Testing the Acrosome Reaction
Acrosome reaction occurs as a result of the release of hydrolyzing enzymes triggered by sperm binding to the zona pellucida. It is a crucial step for successful fertilization as only acrosome-reacted sperm are able to traverse the zona pellucida and fuse with the oolemma [97]. The acrosome of mammalian sperm contains large amounts of acrosin, an essential enzyme for gamete fusion [97]. About 25 percent of men with unexplained infertility were found to have a defective acrosome reaction [98]. Acrosome reaction can be assessed on spermatozoa removed from the surface of the zona pellucida or those exposed to zona pellucida disaggregated proteins using microscopy, flow cytometry, or fluorescently labeled lectins [97].
24.2.5.2 Indications and Clinical Value
Although acrosome reaction testing is more commonly utilized for research purposes, the test could provide some helpful information in couples opting for ART especially when there is prior history of recurrent fertilization failure. Studies have shown that acrosome reaction is a good predictor of fertilization, having a positive predictive value of >75 percent and a negative predictive value of >65 percent [79, 99].
24.2.6 Hyaluronic Binding Assay
24.2.6.1 Rational for Conducting the Hyaluronic Binding Assay
Hyaluronic acid (HA) is a substance that is naturally present around the oocyte acting as a biologic sperm selector [100]. Human sperm contain HA receptors and only mature sperm with normal morphology, minimal DNA fragmentation and chromosomal aneuploidy are able to bind with HA [100]. In other words, the test examines the function of sperm having a normal morphology and an intact acrosome which are more likely to traverse the extracellular matrix, bind to the zona pellucida and fertilize the ovum [101].
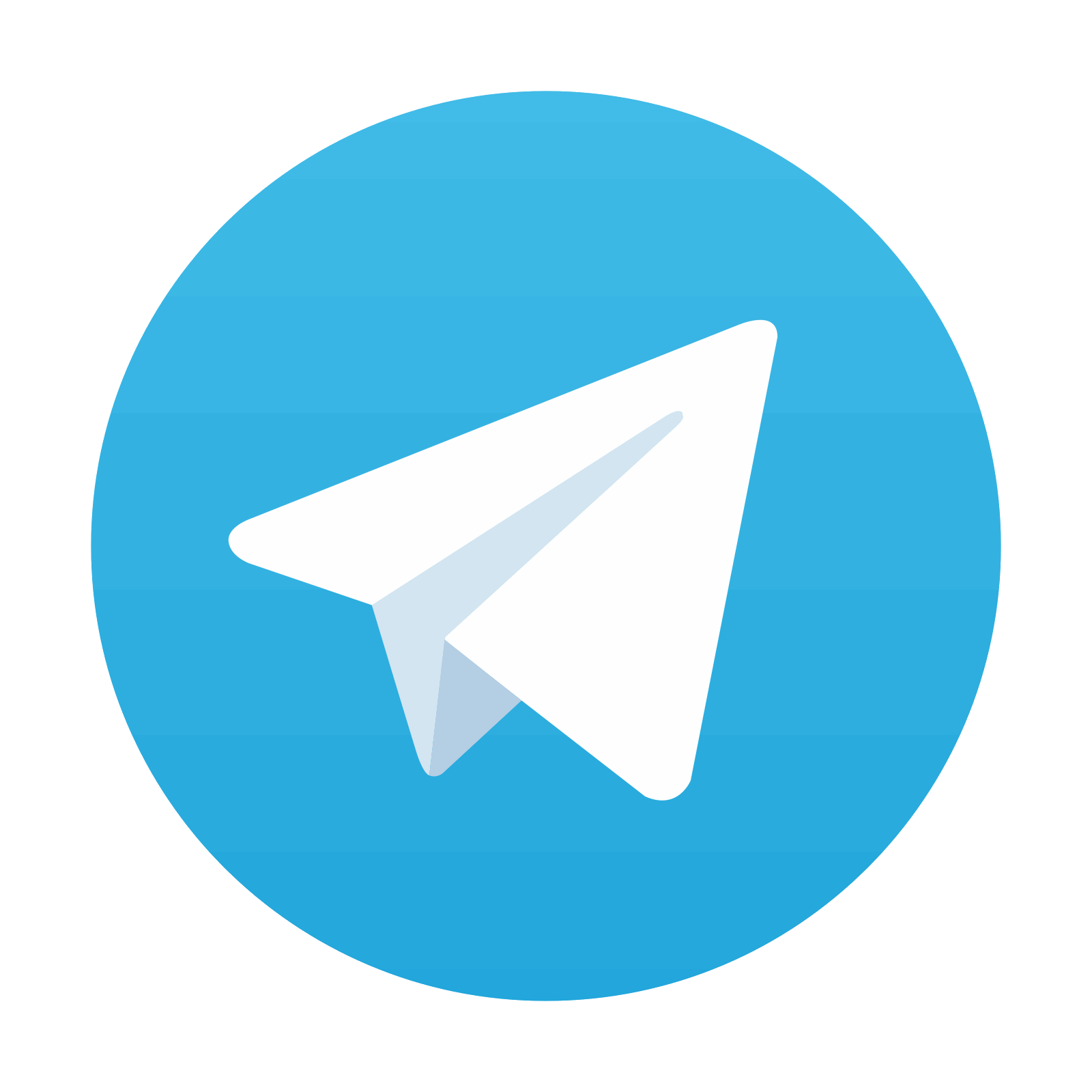
Stay updated, free articles. Join our Telegram channel

Full access? Get Clinical Tree
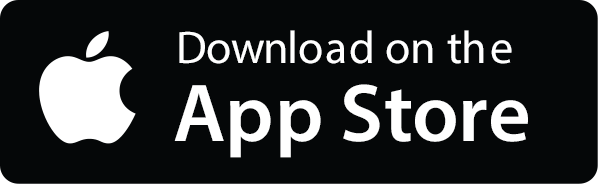
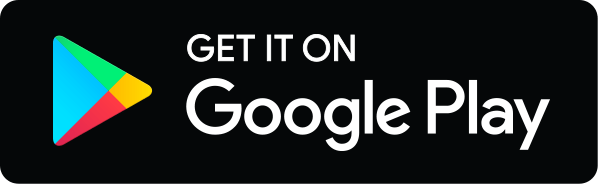
