KEY POINTS
Follow American Burn Association criteria for transfer of a patient to a regional burn center.
Never administer prophylactic antibiotics other than tetanus vaccination.
Early excision and grafting of full-thickness and deep partial-thickness burns improve outcomes.
Intravenous fluid resuscitation for patients with burns greater than 20% of total body surface area (children with burns >15% of total body surface area) should be titrated to mean arterial pressure (MAP) greater than 60 mmHg and urine output greater than 30 mL/h.
INTRODUCTION
Surgical care of the burned patient has evolved into a specialized field incorporating the interdisciplinary skills of burn surgeons, nurses, therapists, and other healthcare specialists. However, recent mass casualty events have been a reminder that healthcare systems may be rapidly pressed to care for large numbers of burn patients. Naturally, general surgeons may be at the forefront in these events, so it is crucial that they are comfortable with the care of burned patients and well equipped to provide standard of care.
BACKGROUND
Burn injury historically carried a poor prognosis. With advances in fluid resuscitation1 and the advent of early excision of the burn wound,2 survival has become an expectation even for patients with severe burns. Continued improvements in critical care and progress in skin bioengineering herald a future in which functional and psychological outcomes are equally important as survival alone. With this shift in priority, the American Burn Association (ABA) has emphasized referral to specialized burn centers after early stabilization. Specific criteria should guide transfer of patients with more complex injuries or other medical needs to a burn center (Table 8-1). The ABA has published standards of care3 and created a verification process to ensure that burn centers meet those standards.4 Because of increased prehospital safety measures, burn patients are being transferred longer distances to receive definitive care at regional burn centers5; recent data from one burn center with a particularly wide catchment area confirmed that even transport times averaging 7 hours did not affect the long-term outcomes of burn patients.6
Partial-thickness burns greater than 10% TBSA |
Burns involving the face, hands, feet, genitalia, perineum, or major joints |
Third-degree burns in any age group |
Electrical burns, including lightning injury |
Chemical burns |
Inhalation injury |
Burn injury in patients with complicated pre-existing medical disorders |
Patients with burns and concomitant trauma in which the burn is the greatest risk. If the trauma is the greater immediate risk, the patient may be stabilized in a trauma center before transfer to a burn center. |
Burned children in hospitals without qualified personnel for the care of children |
Burn injury in patients who will require special social, emotional, or rehabilitative intervention |
INITIAL EVALUATION
Initial evaluation of the burned patient involves four crucial assessments: airway management, evaluation of other injuries, estimation of burn size, and diagnosis of CO and cyanide poisoning. With direct thermal injury to the upper airway or smoke inhalation, rapid and severe airway edema is a potentially lethal threat. Anticipating the need for intubation and establishing an early airway are critical. Perioral burns and singed nasal hairs are signs that the oral cavity and pharynx should be further evaluated for mucosal injury, but these physical findings alone do not indicate an upper airway injury. Signs of impending respiratory compromise may include a hoarse voice, wheezing, or stridor; subjective dyspnea is a particularly concerning symptom and should trigger prompt elective endotracheal intubation. In patients with combined multiple trauma, especially oral trauma, nasotracheal intubation may be useful but should be avoided if oral intubation is safe and easy.
Burned patients should be first considered trauma patients, especially when details of the injury are unclear. A primary survey should be conducted in accordance with Advanced Trauma Life Support guidelines. Concurrently with the primary survey, large-bore peripheral intravenous (IV) catheters should be placed and fluid resuscitation should be initiated; for a burn larger than 40% total body surface area (TBSA), two large-bore IVs are ideal. IV placement through burned skin is safe and effective but requires attention to securing the catheters. Central venous access may provide useful information as to volume status and be useful in severely burned patients. Rarely, IV resuscitation is indicated in patients with burns smaller than 15% who can usually hydrate orally. Pediatric patients with burns larger than 15% may require intraosseous access in emergent situations if venous access cannot be attained. An early and comprehensive secondary survey must be performed on all burn patients, but especially those with a history of associated trauma such as with a motor vehicle collision. Also, patients from structural fires in which the manner of egress is not known should be carefully evaluated for injuries from a possible jump or fall. Urgent radiology studies, such as a chest x-ray, should be performed in the emergency department, but nonurgent skeletal evaluation (i.e., extremity x-rays) can be done in the intensive care unit (ICU) to avoid hypothermia and delays in burn resuscitation. Hypothermia is a common prehospital complication that contributes to resuscitation failure. Patients should be wrapped with clean blankets in transport. Cooling blankets should be avoided in patients with moderate or large (>20% TBSA) burns.
Patients with acute burn injuries should never receive prophylactic antibiotics. This intervention has been clearly demonstrated to promote development of fungal infections and resistant organism and was abandoned in the mid-1980s. A tetanus booster should be administered in the emergency room.
The importance of pain management for these patients has been widely recognized over the past 25 years. However, we must also consider treatment of long-term anxiety. Therefore, it is important to administer an anxiolytic such as a benzodiazepine with the initial narcotics.
Most burn resuscitation formulas estimate fluid requirements using the burn size as a percentage of TBSA (%TBSA). The “rule of nines” is a crude but quick and effective method of estimating burn size (Fig. 8-1). In adults, the anterior and posterior trunk each account for 18%, each lower extremity is 18%, each upper extremity is 9%, and the head is 9%. In children under 3 years old, the head accounts for a larger relative surface area and should be taken into account when estimating burn size. Diagrams such as the Lund and Browder chart give a more accurate accounting of the true burn size in children. The importance of an accurate burn size assessment cannot be overemphasized. Superficial or first-degree burns should not be included when calculating the %TBSA, and thorough cleaning of soot and debris is mandatory to avoid confusing soiled skin with burns. Examination of referral data suggests that physicians inexperienced with burns tend to overestimate the size of small burns and underestimate the size of large burns, with potentially detrimental effects on pretransfer resuscitation.7
An important contributor to early mortality in burn patients is carbon monoxide (CO) poisoning resulting from smoke inhalation. The affinity of CO for hemoglobin is approximately 200 to 250 times more than that of oxygen, which decreases the levels of normal oxygenated hemoglobin and can quickly lead to anoxia and death.8 Unexpected neurologic symptoms should raise the level of suspicion, and an arterial carboxyhemoglobin level must be obtained because pulse oximetry can be falsely elevated. Administration of 100% oxygen is the gold standard for treatment of CO poisoning and reduces the half-life of CO from 250 minutes in room air to 40 to 60 minutes on 100% oxygen.9 Some authors have proposed hyperbaric oxygen as an adjunctive therapy for CO poisoning.10 However, the data are mixed regarding the success of hyperbaric oxygen, and its associated logistical difficulties and complications have limited its usefulness for patients with moderate or large burns.11,12 Patients who sustain a cardiac arrest as a result of their CO poisoning have an extremely poor prognosis regardless of the success of initial resuscitation attempts.13 Hydrogen cyanide toxicity may also be a component of smoke inhalation injury. Afflicted patients may have a persistent lactic acidosis or ST elevation on electrocardiogram (ECG).14 Cyanide inhibits cytochrome oxidase, which is required for oxidative phosphorylation.15 Treatment consists of sodium thiosulfate, hydroxocobalamin, and 100% oxygen. Sodium thiosulfate works by transforming cyanide into a nontoxic thiocyanate derivative, but it works slowly and is not effective for acute therapy. Hydroxocobalamin quickly complexes with cyanide, is excreted by the kidney, and is recommended for immediate therapy.9 In the majority of patients, the lactic acidosis will resolve with ventilation, and sodium thiosulfate treatment becomes unnecessary.16
CLASSIFICATION OF BURNS
Burns are commonly classified as thermal, electrical, or chemical burns, with thermal burns consisting of flame, contact, or scald burns. Flame burns are not only the most common cause for hospital admission of burns, but also have the highest mortality. This is primarily related to their association with structural fires and the accompanying inhalation injury and/or CO poisoning.17
Electrical burns make up only 4% of U.S. hospital admissions but have special concerns including the potential for cardiac arrhythmias and compartment syndromes with concurrent rhabdomyolysis. A baseline ECG is recommended in all patients with an electrical injury, and a normal ECG in a low-voltage injury may preclude hospital admission. Because compartment syndrome and rhabdomyolysis are common in high-voltage electrical injuries, vigilance must be maintained for neurologic or vascular compromise, and fasciotomies should be performed even in cases of moderate clinical suspicion. Long-term neurologic and visual symptoms are not uncommon with high-voltage electrical injuries, and ophthalmologic and neurologic consultation should be obtained to better define a patient’s baseline function.18
Chemical burns are less common but potentially severe burns. The most important components of initial therapy are careful removal of the toxic substance from the patient and irrigation of the affected area with water for a minimum of 30 minutes, except in cases of concrete powder or powdered forms of lye, which should be swept from the patient to avoid activating the aluminum hydroxide with water. The offending agents in chemical burns can be systemically absorbed and may cause specific metabolic derangements. Formic acid has been known to cause hemolysis and hemoglobinuria, and hydrofluoric acid causes hypocalcemia. Hydrofluoric acid is a particularly common offender due to its widespread industrial uses. Calcium-based therapies are the mainstay of treating hydrofluoric acid burns, with topical application of calcium gluconate onto wounds19 and IV administration of calcium gluconate for systemic symptoms. Intra-arterial calcium gluconate infusion provides effective treatment of progressive tissue injury and intense pain.20,21 Patients undergoing intra-arterial therapy need continuous cardiac monitoring. Persistent refractory hypocalcemia with electrocardiac abnormalities may signal the need for emergent excision of the burned areas.
BURN DEPTH
Based on the original burn depth classification by Dupuytren in 1832,22 burn wounds are commonly classified as superficial (first-degree), partial-thickness (second-degree), full-thickness (third-degree), and fourth-degree burns, which affect underlying soft tissue. Partial-thickness burns are classified as either superficial or deep partial-thickness burns by depth of involved dermis. Clinically, first-degree burns are painful but do not blister, second-degree burns have dermal involvement and are extremely painful with weeping and blisters, and third-degree burns are leathery, painless, and nonblanching. Jackson described three zones of tissue injury following burn injury.23 The zone of coagulation is the most severely burned portion and is typically in the center of the wound. As the name implies, the affected tissue is coagulated and sometimes frankly necrotic, much like a third- or fourth-degree burn, and will need excision and grafting. Peripheral to that is a zone of stasis, with variable degrees of vasoconstriction and resultant ischemia, much like a second-degree burn. Appropriate resuscitation and wound care may help prevent conversion to a deeper wound, but infection or suboptimal perfusion may result in an increase in burn depth. This is clinically relevant because many superficial partial-thickness burns will heal with expectant management, and the majority of deep partial-thickness burns require excision and skin grafting. The last area of a burn is called the zone of hyperemia, which will heal with minimal or no scarring and is most like a superficial or first-degree burn.
Unfortunately, even experienced burn surgeons have limited ability to accurately predict the healing potential of partial-thickness burns soon after injury; one reason is that burn wounds evolve over the 48 to 72 hours after injury. Numerous techniques have been developed with the idea that better early prediction of burn depth will expedite appropriate surgical decision making. One of the most effective ways to determine burn depth is full-thickness biopsy, but this has several limitations; not only is the procedure painful and potentially scarring, but accurate interpretation of the histopathology requires a specialized pathologist and may have slow turnaround times.24 Laser Doppler can measure skin perfusion to predict burn depth with a positive predictive value of up to 80% in some studies.25,26 Noncontact ultrasound has been postulated as a painless modality to predict nonhealing wounds and has the advantage of easily performed serial measurements.27 Unfortunately, none of these newer therapies have proven adequately superior to justify their cost and as yet have not substituted serial examination by experienced burn surgeons.
PROGNOSIS
The Baux score (mortality risk equals age plus %TBSA) was used for many years to predict mortality in burns. Analysis of multiple risk factors for burn mortality has validated age and %TBSA as the strongest predictors of mortality.28 Advancements in burn care have lowered overall mortality to the point that the Baux score may no longer be accurate. However, age and burn size, as well as inhalation injury, continue to be the most robust indicators for burn mortality.29 Age even as a single variable strongly predicts mortality in burns,30 and in-hospital mortality in elderly burn patients is a function of age regardless of other comorbidities.31 In nonelderly patients, comorbidities such as preinjury human immunodeficiency virus (HIV), metastatic cancer, and kidney or liver disease may influence mortality and length of stay.32 A recent large database study of 68,661 burn patients found that the variables with the highest predictive value for mortality were age, %TBSA, inhalation injury, coexistent trauma, and pneumonia.33
RESUSCITATION
A myriad of formulas exist for calculating fluid needs during burn resuscitation, suggesting that no one formula benefits all patients. The most commonly used formula, the Parkland or Baxter formula, consists of 3 to 4 mL/kg/% burn of lactated Ringer’s, of which half is given during the first 8 hours after burn and the remaining half is given over the subsequent 16 hours. The concept behind continuous fluid requirements is simple. The burn (and/or inhalation injury) drives an inflammatory response that leads to capillary leak; as plasma leaks into the extravascular space, crystalloid administration maintains the intravascular volume. Therefore, if a patient receives a large fluid bolus in a prehospital setting or emergency department, that fluid has likely leaked into the interstitium and the patient still requires ongoing burn resuscitation according to the estimates. Continuation of fluid volumes should depend on the time since injury, urine output, and mean arterial pressure (MAP). As the leak closes, the patient will require less volume to maintain these two resuscitation endpoints. Children under 20 kg have the additional requirement that they do not have sufficient glycogen stores to maintain an adequate glucose level in response to the inflammatory response. Specific pediatric formulas have been described, but the simplest approach is to deliver a weight-based maintenance IV fluid with glucose supplementation in addition to the calculated resuscitation fluid with lactated Ringer’s.
It is important to remember that any formula for burn resuscitation is merely a guideline, and fluid must be titrated based on appropriate measures of adequate resuscitation. A number of parameters are widely used to gauge burn resuscitation, but the most common remain the simple outcomes of blood pressure and urine output. As in any critically ill patient, a target MAP of 60 mmHg ensures optimal end-organ perfusion. Goals for urine output should be 30 mL/h in adults and 1 to 1.5 mL/kg/h in pediatric patients. Because blood pressure and urine output may not correlate perfectly with true tissue perfusion, the search continues for other adjunctive parameters that may more accurately reflect adequate resuscitation. Some centers have found serum lactate to be a better predictor of mortality in severe burns,34,35 and others have found that base deficit predicts eventual organ dysfunction and mortality.36,37 Because burned patients with normal blood pressure and serum lactate levels may have compromised gastric mucosal perfusion, continuous measurement of mucosal pH with its logistical difficulties has garnered limited popularity.38,39 Invasive monitoring with pulmonary artery catheters typically results in significant excessive fluid administration without improved cardiac output or preload measurements; use of invasive monitoring seems to have variable effects on long-term outcomes.40
Actual administrated fluid volumes typically exceed volumes predicted by standard formulas.41 One survey of burn centers showed that 58% of patients end up getting more fluids than would be predicted by Baxter’s formula.42 Comparison of modern-day patients with historical controls shows that over-resuscitation may be a relatively recent trend.43 One theory is that increased opioid analgesic use results in peripheral vasodilation and hypotension and the need for greater volumes of bloused resuscitative fluids.44 A classic study by Navar et al showed that burned patients with inhalation injury required an average of 5.76 mL/kg/% burn, vs. 3.98 mL/kg/% burn for patients without inhalation injury, and this has been corroborated by subsequent studies.45,46 Prolonged mechanical ventilation may also play a role in increased fluid needs.47 A recent multicenter study found that age, weight, %TBSA, and intubation on admission were significant predictors of more fluid delivery during the resuscitation period. Those patients receiving higher fluid volumes were at increased risk of complications and death.48 Common complications include abdominal compartment syndrome, extremity compartment syndrome, intraocular compartment syndrome, and pleural effusions. Monitoring bladder pressures can provide valuable information about development of intra-abdominal hypertension.
The use of colloid as part of the burn resuscitation has generated much interest over the years. In late resuscitation when the capillary leak has closed, colloid administration may decrease overall fluid volumes and potentially may decrease associated complications such as intra-abdominal hypertension.49 However, albumin use has never been shown to improve outcomes in burn patients and has controversial effects on mortality in critically ill patients.50,51 Attempts to minimize fluid volumes in burn resuscitation have included study of hypertonic solutions, which appear to transiently decrease initial resuscitation volumes, with the downside of causing hyperchloremic acidosis.52
Other adjuncts are being increasingly used during initial burn resuscitation. High-dose ascorbic acid (vitamin C) may decrease fluid volume requirements and ameliorate respiratory embarrassment during resuscitation.53 Plasmapheresis may also decrease fluid requirements in patients who require higher volumes than predicted to maintain adequate urine output and MAP. It is postulated that plasmapheresis may filter out inflammatory mediators, thus decreasing ongoing vasodilation and capillary leak.54
One recent adjunct that has found increasing utility in other surgical ICUs has been the application of bedside thoracic ultrasound.55 Ultrasound offers the potential to make rapid, noninvasive assessments during acute changes in clinical condition. For burn patients, bedside ultrasonography may be indicated for evaluation of volume status, gross assessment of cardiac function, and diagnosis of pneumothorax. Determining patient cardiac function and volume status may guide fluid resuscitation. Cardiac function can be evaluated with three common heart views: the parasternal long axis, parasternal short axis, and apical four-chamber views.56 Volume status can be estimated by examination of cardiac function, evaluation of the inferior vena cava (IVC) diameter, and changes with respiration. Ultrasound also allows timely diagnosis of pneumothorax.57 A high-frequency probe with an adequate window between ribs permits identification of lung parenchyma against the chest well. A pneumothorax appears as a transition on ultrasound between lung parenchyma, which has a heterogeneous appearance, and air, which has a hypoechoic appearance. Further studies are warranted to identify indications for the use of ultrasound in burned patients.
TRANSFUSION
The role of blood transfusion in critically injured patients has undergone a reevaluation in recent years.58,59 Blood transfusions are considered to be immunosuppressive, which is one explanation for the common responses seen to blood transfusions, such as increased infection and shorter time to recurrence after oncologic surgery.60 A large multicenter study of blood transfusions in burn patients found that increased numbers of transfusions were associated with increased infections and higher mortality in burn patients, even when correcting for burn severity.61 A follow-up study implanting a restrictive transfusion policy in burned children showed that a hemoglobin threshold of 7 g/dL had no more adverse outcomes vs. a traditional transfusion trigger of 10 g/dL. In addition, costs incurred to the institution were significantly less.62 These data, in concert with other reported complications such as transfusion-related lung injury,63 have led to recommendations that blood transfusions be used only when there is an apparent physiologic need. Attempts to minimize blood transfusion in nonburned critically ill patients have led to use of erythropoietin by some centers. However, burn patients often have elevated erythropoietin levels, and a randomized study in burn patients showed that recombinant human erythropoietin did not effectively prevent anemia or decrease the number of transfusions given.64
INHALATION INJURY AND VENTILATOR MANAGEMENT
Inhalation injuries are commonly seen in tandem with burn injuries and are known to increase mortality in burned patients.65 Smoke inhalation is present in as many as 35% of hospitalized burn patients and may triple the hospital stay compared to isolated burn injuries.66 The combination of burns, inhalation injury, and pneumonia increases mortality by up to 60% over burns alone.67 Subsequent development of the adult respiratory distress syndrome (ARDS) is common in these patients and may be caused in part by recruitment of alveolar leukocytes with an enhanced endotoxin-activated cytokine response.68 When ARDS complicates burns and inhalation injury, mortality approaches 66%; in one study, patients with burns ≥60% TBSA in combination with inhalation injury and ARDS had 100% mortality.69
Smoke inhalation causes injury in two ways: by direct heat injury to the upper airways and inhalation of combustion products into the lower airways. Direct injury to the upper airway causes airway swelling that typically leads to maximal edema in the first 24 to 48 hours after injury and often requires a short course of endotracheal intubation for airway protection. Combustion products found in smoke, most commonly from synthetic substances in structural fires, cause lower airway injury. These irritants cause direct mucosal injury, which in turn leads to mucosal sloughing, edema, reactive bronchoconstriction, and finally obstruction of the lower airways. Injury to both the epithelium and pulmonary alveolar macrophages causes release of prostaglandins, chemokines, and other inflammatory mediators; neutrophil migration; increased tracheobronchial blood flow; and finally increased capillary permeability. All of these components of acute lung injury increase the risk of pneumonia and ARDS following an inhalation injury.
The physiologic effects of smoke inhalation are numerous. Inhalation injury decreases lung compliance70 and increases airway resistance work of breathing.71 Inhalation injury in the presence of burns also increases overall metabolic demands.72 The most common physiologic derangement seen with inhalation injury is increased fluid requirement during resuscitation. Since severe inhalation injury may result in mucosal sloughing with obstruction of smaller airways, bronchoscopy findings including carbon deposits, erythema, edema, bronchorrhea, and a hemorrhagic appearance may be useful for staging inhalation injury. Furthermore, bronchoalveolar lavage within 24 hours after an inhalation injury demonstrates a high rate of positive quantitative cultures,73 suggesting that pneumonia develops soon after the acute lung injury. Because bronchoscopy is an invasive test, attempts have been made to utilize other diagnostic modalities, such as thoracic computed tomography (CT) scans74 and xenon ventilation-perfusion scanning.75 Decreased Pao2:Fio2 ratio (<200) on admission may not only predict inhalation injury but also indicate increased fluid needs more accurately than bronchoscopic grading of the severity of inhalation.76
Treatment of inhalation injury consists primarily of supportive care. Aggressive pulmonary toilet and routine use of nebulized bronchodilators such as albuterol are recommended. Nebulized N-acetylcysteine is an antioxidant free radical scavenger designed to decrease the toxicity of high oxygen concentrations. Aerosolized heparin aims to prevent formation of fibrin plugs and decrease the formation of airway casts. These agents seem to improve pulmonary toilet but have no demonstrated effect on mortality.77 Aerosolized tissue plasminogen activator78 and recombinant human antithrombin79 have shown promise in sheep models, but have not yet seen widespread clinical use. Administration of intrabronchial surfactant has been used as a salvage therapy in patients with severe burns and inhalation injury.80 Inhaled nitric oxide may also be useful as a last effort in burn patients with severe lung injury who are failing other means of ventilatory support.81 The use of steroids has traditionally been avoided due to worse outcomes in burn patients,82 but new promising data in late ARDS have prompted scientific review of steroid use.83
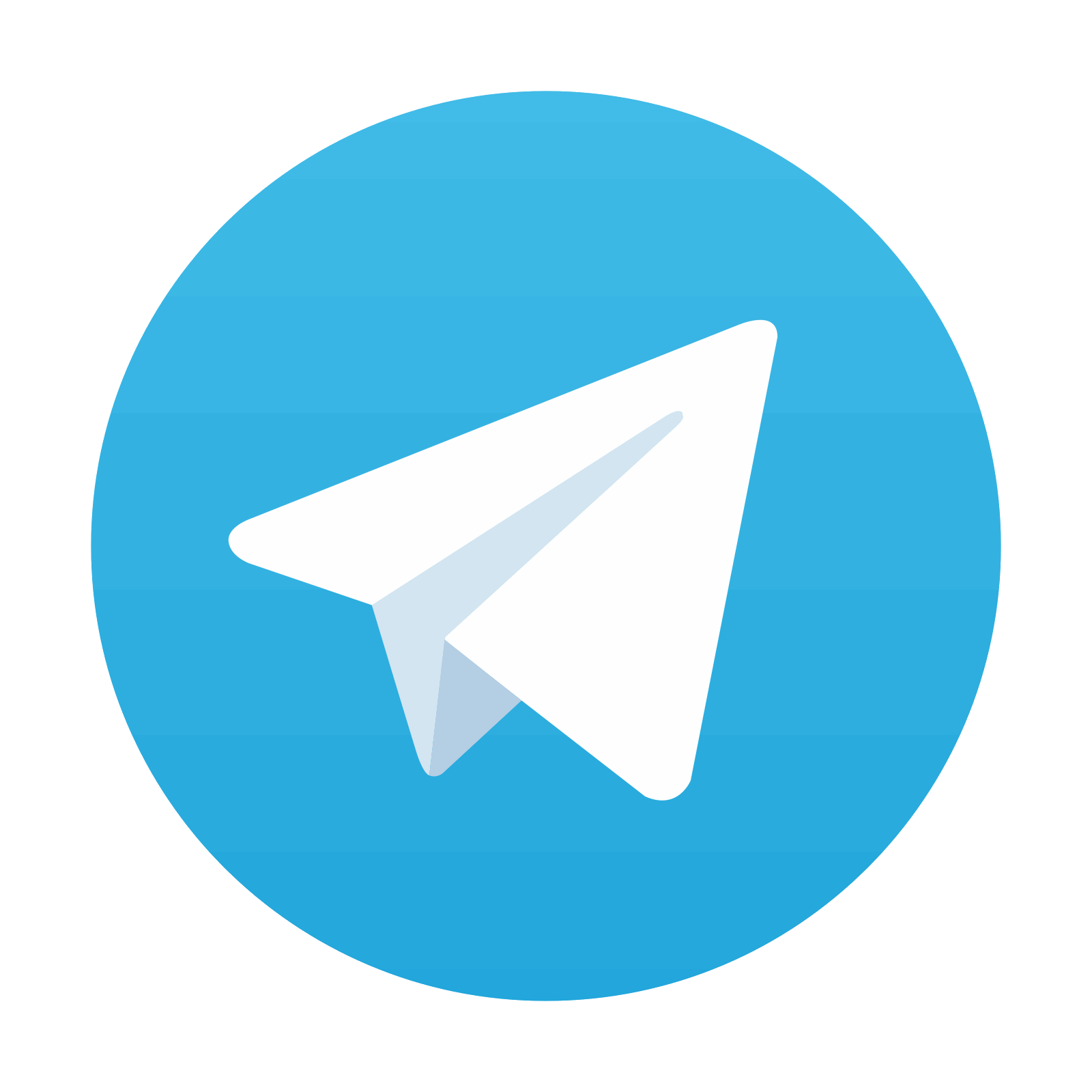
Stay updated, free articles. Join our Telegram channel

Full access? Get Clinical Tree
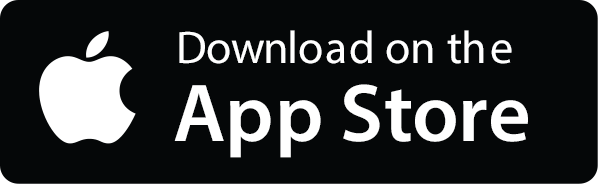
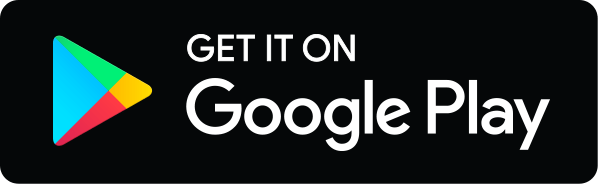