KEY POINTS
Carotid intervention as a preventive strategy should be performed in patients with 50% or greater symptomatic internal carotid artery stenosis and those with 80% or greater asymptomatic internal carotid artery stenosis. Carotid intervention for asymptomatic stenosis between 60% and 79% remains controversial and is a function of an operator’s stroke rate. The choice of intervention—carotid endarterectomy versus carotid stenting—remains controversial; currently, carotid endarterectomy appears to be associated with lower stroke rate, whereas carotid stenting is more suitable under certain anatomic or physiologic conditions.
Abdominal aortic aneurysms should be repaired when the risk of rupture, determined mainly by aneurysm size, exceeds the risk of death due to perioperative complications or concurrent illness. Endovascular repair is associated with less perioperative morbidity and mortality compared to open reconstruction and is preferred for high-risk patients who meet specific anatomic criteria.
Symptomatic mesenteric ischemia should be treated to improve quality of life and prevent bowel infarction. Operative treatment—bypass—is superior to endovascular intervention, although changes in wire and stent technology have improved the results of mesenteric stenting in recent series.
Aortoiliac occlusive disease can be treated with either endovascular means or open reconstruction, depending on patient risk stratification, occlusion characteristics, and symptomatology.
Claudication is a marker of extensive atherosclerosis and is mainly managed with risk factor modification and pharmacotherapy. Only 5% of patients with claudication will need intervention because of disabling extremity pain. The 5-year mortality of a patient with claudication approaches 30%. Patients with rest pain or tissue loss need expeditious evaluation and vascular reconstruction to ameliorate the severe extremity pain and prevent limb loss.
GENERAL APPROACH TO THE VASCULAR PATIENT
Since the vascular system involves every organ system in our body, the symptoms of vascular disease are as varied as those encountered in any medical specialty. Lack of adequate blood supply to target organs typically presents with pain; for example, calf pain with lower extremity claudication, postprandial abdominal pain from mesenteric ischemia, and arm pain with axillo-subclavian arterial occlusion. In contrast, stroke and transient ischemic attack (TIA) are the presenting symptoms from middle cerebral embolization as a consequence of a stenosed internal carotid artery. The pain syndrome of arterial disease is usually divided clinically into acute and chronic types, with all shades of severity between the two extremes. Sudden onset of pain can indicate complete occlusion of a critical vessel, leading to more severe pain and critical ischemia in the target organ, resulting in lower limb gangrene or intestinal infarction. Chronic pain results from a slower, more progressive atherosclerotic occlusion, which can be totally or partially compensated by developing collateral vessels. Acute on chronic is another pain pattern in which a patient most likely has an underlying arterial stenosis that suddenly occludes; for example, the patient with a history of calf claudication who now presents with sudden, severe acute limb-threatening ischemia. The clinician should always try to understand and relate the clinical manifestations to the underlying pathologic process.
Appropriate history should be focused based on the presenting symptoms related to the vascular system (Table 23-1). Of particular importance in the previous medical history is noting prior vascular interventions (endovascular or open surgical), and all vascular patients should have inquiry made about their prior cardiac history and current cardiac symptoms. Approximately 30% of vascular patients will be diabetic. A history of prior and current smoking status should be noted.
• History of stroke or transient ischemic attack • History of coronary artery disease, including previous myocardial infarction and angina • History of peripheral arterial disease • History of diabetes • History of hypertension • History of tobacco use • History of hyperlipidemia |
The patient with carotid disease in most cases is completely asymptomatic, having been referred based on the finding of a cervical bruit or duplex finding of stenosis. Symptoms of carotid territory TIAs include transient monocular blindness (amaurosis), contralateral weakness or numbness, and dysphasia. Symptoms persisting longer than 24 hours constitute a stroke. In contrast, the patient with chronic mesenteric ischemia is likely to present with postprandial abdominal pain and weight loss. The patient fears eating because of the pain, avoids food, and loses weight. It is very unlikely that a patient with abdominal pain who has not lost weight has chronic mesenteric ischemia.
The patient with lower extremity pain on ambulation has intermittent claudication that occurs in certain muscle groups; for example, calf pain upon exercise usually reflects superficial femoral artery disease, while pain in the buttocks reflects iliac disease. In most cases, the pain manifests in one muscle group below the level of the affected artery, occurs only with exercise, and is relieved with rest only to recur at the same location, hence the term “window gazer’s disease.” Rest pain (a manifestation of severe underlying occlusive disease) is constant and occurs in the foot (not the muscle groups), typically at the metatarsophalangeal junction, and is relieved by dependency. Often the patient is prompted to sleep with their foot hanging off one side of the bed to increase the hydrostatic pressure.
Specific vascular examination should include abdominal aortic palpation, carotid artery examination, and pulse examination of the lower extremity (femoral, popliteal, posterior tibial, and dorsalis pedis arteries). The abdomen should be palpated for an abdominal aortic aneurysm, detected as an expansile pulse above the level of the umbilicus. It should also be examined for the presence of bruits. Because the aorta typically divides at the level of the umbilicus, an aortic aneurysm is most frequently palpable in the epigastrium. In thin individuals, a normal aortic pulsation is palpable, while in obese patients, even large aortic aneurysms may not be detectable. Suspicion of a clinically enlarged aorta should lead to the performance of an ultrasound scan for a more accurate definition of aortic diameter.
The carotids should be auscultated for the presence of bruits, although there is a higher correlation with coronary artery disease than underlying carotid stenosis. A bruit at the angle of the mandible is a significant finding, leading to follow-up duplex scanning. The differential diagnosis is a transmitted murmur from a sclerotic or stenotic aortic valve. The carotid is palpable deep to the sternocleidomastoid muscle in the neck. Palpation, however, should be gentle and rarely yields clinically useful information.
Upper extremity examination is necessary when an arteriovenous graft is to be inserted in patients who have symptoms of arm pain with exercise. Thoracic outlet syndrome (TOS) can result in occlusion or aneurysm formation of the subclavian artery. Distal embolization is a manifestation of TOS; consequently, the fingers should be examined for signs of ischemia and ulceration. The axillary artery enters the limb below the middle of the clavicle, where it can be palpated in thin patients. It is usually easily palpable in the axilla and medial upper arm. The brachial artery is most easily located at the antecubital fossa immediately medial to the biceps tendon. The radial artery is palpable at the wrist anterior to the radius.
For lower extremity vascular examination, the femoral pulse is usually palpable midway between the anterior superior iliac spine and the pubic tubercle. The popliteal artery is palpated in the popliteal fossa with the knee flexed to 45° and the foot supported on the examination table to relax the calf muscles. Palpation of the popliteal artery is a bimanual technique. Both thumbs are placed on the tibial tuberosity anteriorly and the fingers are placed into the popliteal fossa between the two heads of the gastrocnemius muscle. The popliteal artery is palpated by compressing it against the posterior aspect of the tibia just below the knee. The posterior tibial pulse is detected by palpation 2 cm posterior to the medial malleolus. The dorsalis pedis is detected 1 cm lateral to the hallucis longus extensor tendon, which dorsiflexes the great toe and is clearly visible on the dorsum of the foot. Pulses can be graded using either the traditional four-point scale or the basic two-point scale system (Table 23-2). The foot should also be carefully examined for pallor on elevation and rubor on dependency, as these findings are indicative of chronic ischemia. Note should also be made of nail changes and loss of hair. Ulceration and other findings specific to disease states are described in relevant sections later in this chapter.
After reconstructive vascular surgery, the graft may be available for examination, depending on its type and course. The in situ lower extremity graft runs in the subcutaneous fat and can be palpated along most of its length. A change in pulse quality, aneurysmal enlargement, or a new bruit should be carefully noted. Axillofemoral grafts, femoral-to-femoral grafts, and arteriovenous access grafts can usually be easily palpated as well.
There is increasing interest in the use of the ankle-brachial index (ABI) to evaluate patients at risk for cardiovascular events. An ABI less than 0.9 correlates with increased risk of myocardial infarction and indicates significant, although perhaps asymptomatic, underlying peripheral vascular disease. The ABI is determined in the following ways. Blood pressure is measured in both upper extremities using the highest systolic blood pressure as the denominator for the ABI. The ankle pressure is determined by placing a blood pressure cuff above the ankle and measuring the return to flow of the posterior tibial and dorsalis pedis arteries using a pencil Doppler probe over each artery. The ratio of the systolic pressure in each vessel divided by the highest arm systolic pressure can be used to express the ABI in both the posterior tibial and dorsalis pedis arteries (Fig. 23-1). Normal is more than 1. Patients with claudication typically have an ABI in the 0.5 to 0.7 range, and those with rest pain are in the 0.3 to 0.5 range. Those with gangrene have an ABI of less than 0.3. These ranges can vary depending on the degree of compressibility of the vessel. The test is less reliable in patients with heavily calcified vessels. Due to noncompressibility, some patients, such as diabetics and those with end-stage renal disease, may have ABI ≥1.40 and require additional noninvasive diagnostic testing to evaluate for peripheral artery disease. Alternative tests include toe-brachial pressures, pulse volume recordings, transcutaneous oxygen measurements, or vascular imaging (duplex ultrasound).
By placing serial blood pressure cuffs down the lower extremity and then measuring the pressure with a Doppler probe as flow returns to the artery below the cuff, it is possible to determine segmental pressures down the leg. This data can then be used to infer the level of the occlusion. The systolic pressure at each level is expressed as a ratio, with the highest systolic pressure in the upper extremities as the denominator. Normal segmental pressures commonly show high thigh pressures 20 mmHg or greater in comparison to the brachial artery pressures. The low thigh pressure should be equivalent to brachial pressures. Subsequent pressures should fall by no more than 10 mmHg at each level. A pressure gradient of 20 mmHg between two subsequent levels is usually indicative of occlusive disease at that level. The most frequently used index is the ratio of the ankle pressure to the brachial pressure, the ABI. Normally, the ABI is greater than 1.0, and a value of less than 0.9 indicates some degree of arterial obstruction and has been shown to be correlated with an increased risk of coronary heart disease.1 Limitations of relying on segmental limb pressures include: (a) missing isolated moderate stenoses (usually iliac) that produce little or no pressure gradient at rest; (b) falsely elevated pressures in patients with diabetes and end-stage renal disease; and (c) the inability to differentiate between stenosis and occlusion.2 Patients with diabetes and end-stage renal disease have calcified vessels that are difficult to compress, thus rendering this method inaccurate, due to recording of falsely elevated pressure readings. Noncompressible arteries yield ankle systolic pressures ≥250 mmHg and ABIs >1.40. In this situation, absolute toe and ankle pressures can be measured to gauge critical limb ischemia. Ankle pressures less than 50 mmHg or toe pressures less than 30 mmHg are indicative of critical limb ischemia. The toe pressure is normally 30 mmHg less than the ankle pressure, and a toe-brachial index (TBI) <0.70 is abnormal. False-positive results with the TBI are unusual. The main limitation of this technique is that it may be impossible to measure pressures in the first and second toes due to pre-existing ulceration.
In patients with noncompressible vessels, segmental plethysmography can be used to determine underlying arterial occlusive disease. Cuffs placed at different levels on the leg detect changes in blood volume and produce a pulse volume recording (PVR) when connected to a plethysmograph (Fig. 23-2). To obtain accurate PVR waveforms, the cuff is inflated to 60 to 65 mmHg, so as to detect volume changes without causing arterial occlusion. Pulse volume tracings are suggestive of proximal disease if the upstroke of the pulse is not brisk, the peak of the wave tracing is rounded, and there is disappearance of the dicrotic notch.
Although isolated segmental limb pressures and PVR measurements are 85% accurate when compared with angiography in detecting and localizing significant atherosclerotic lesions, when used in combination, accuracy approaches 95%.3 For this reason, it is suggested that these two diagnostic modalities be used in combination when evaluating peripheral artery disease.
Ultrasound examinations are relatively time consuming, require experienced technicians, and may not visualize all arterial segments. Doppler waveform analysis can suggest atherosclerotic occlusive disease if the waveforms in the insonated arteries are biphasic, monophasic, or asymmetrical. B-mode ultrasonography provides black and white, real-time images. B-mode ultrasonography does not evaluate blood flow; thus, it cannot differentiate between fresh thrombus and flowing blood, which have the same echogenicity. Calcification in atherosclerotic plaques will cause acoustic shadowing. B-mode ultrasound probes cannot be sterilized. Use of the B-mode probe intraoperatively requires a sterile covering and gel to maintain an acoustic interface. Experience is needed to obtain and interpret images accurately. Duplex ultrasonography entails performance of B-mode imaging, spectral Doppler scanning, and color-flow duplex scanning. The caveat to performance of duplex ultrasonography is meticulous technique by a certified vascular ultrasound technician, so that the appropriate 60° Doppler angle is maintained during insonation with the ultrasound probe. Alteration of this angle can markedly alter waveform appearance and subsequent interpretation of velocity measurements. Direct imaging of intra-abdominal vessels with duplex ultrasound is less reliable because of the difficulty in visualizing the vessels through overlying bowel. These disadvantages currently limit the applicability of duplex scanning in the evaluation of aortoiliac and infrapopliteal disease. In a recent study, duplex ultrasonography had lower sensitivity in the calculation of infrapopliteal vessel stenosis in comparison to conventional digital subtraction or computed tomography angiography.4 Few surgeons rely solely on duplex ultrasonography for preoperative planning in lower extremity revascularizations; but with experience, lower extremity arteries can be insonated to determine anatomy, and the functional significance of lesions can be determined by calculation of degree of stenosis from velocity ratios. Duplex scanning is unable to evaluate recently implanted polytetrafluoroethylene (PTFE) and polyester (Dacron) grafts because they contain air, which prevents ultrasound penetration.
Computed tomography angiography (CTA) is a noninvasive, contrast-dependent method for imaging the arterial system. It depends on intravenous infusion of iodine-based contrast agents. The patient is advanced through a rotating gantry, which images serial transverse slices. The contrast-filled vessels can be extracted from the slices and rendered in three-dimensional format (Fig. 23-3). The extracted images can also be rotated and viewed from several different directions during postacquisition image processing. This technology has been advanced as a consequence of aortic endografting. CTA provides images for postprocessing that can be used to display the aneurysm in a format that demonstrates thrombus, calcium, lumen, and the outer wall, and allows “fitting” of a proposed endograft into the aneurysm (Fig. 23-4). CTA is increasingly being used to image the carotid bifurcation, and as computing power increases, the speed of image acquisition and resolution will continue to increase. The major limitations of multidetector CTA are use of contrast and presence of artifacts caused by calcification and stents. CTA can overestimate the degree of in-stent stenosis, while heavy calcification can limit the diagnostic accuracy of the method by causing a “blooming artifact.”5 The artifacts can be overcome with alteration in image acquisition technique. There are no randomized trials to document the superiority of multidetector CTA over traditional angiography, but there is emerging evidence to support the claim that multidetector CTA has sensitivity, specificity, and accuracy that rival invasive angiography.5
Figure 23-3.
A multidetector computed tomography angiography with three-dimensional reconstruction of the iliofemoral arterial circulation in two patients with lower leg claudication. A. A 50-year-old male with an occluded right superficial femoral artery (single long arrow) with reconstituted superficial femoral artery at the level of mid-thigh. Arterial calcifications (single short arrows) are present in the bilateral distal superficial femoral arteries. B. A 53-year-old male with occluded right common iliac artery (double arrows).
Magnetic resonance angiography (MRA) has the advantage of not requiring iodinated contrast agents to provide vessel opacification (Fig. 23-5). Gadolinium is used as a contrast agent for MRA studies, and because it is generally not nephrotoxic, it can be used in patients with elevated creatinine. MRA is contraindicated in patients with pacemakers, defibrillators, spinal cord stimulators, intracerebral shunts, cochlear implants, and cranial clips. Patients with claustrophobia may require sedation to be able to complete the test. The presence of metallic stents causes artifacts and signal drop-out; however, these can be dealt with using alternations in image acquisition and processing. Nitinol stents produce minimal artifact.6 Compared to other modalities, MRA is relatively slow and expensive. However, due to its noninvasive nature and decreased nephrotoxicity, MRA is being used more frequently for imaging vasculature in various anatomic distributions.
Diagnostic angiography is considered the gold standard in vascular imaging. In many centers, its use is rapidly decreasing due to the development of noninvasive imaging modalities such as duplex arterial mapping, CTA, and MRA. Nevertheless, contrast angiography still remains in widespread use. The essential aspects of angiography are vascular access and catheter placement in the vascular bed that requires examination. The imaging system and the contrast agent are used to opacify the target vessel. Although in the past this function has largely been delegated to the interventional radiology service, an increasing number of surgeons are performing this procedure and following the diagnostic imaging with immediate surgical or endovascular intervention. There are several considerations when relying on angiography for imaging.
Approximately 70% of atherosclerotic plaques occur in an eccentric location within the blood vessel; therefore, images can be misleading when trying to evaluate stenoses because angiography is limited to a uniplanar “lumenogram.” With increased use of intravascular stent deployment, it has also been noted that assessment of stent apposition and stent position in relation to surrounding branches may be inaccurate. Furthermore, angiography exposes the patient to the risks of both ionizing radiation and intravascular contrast. Nevertheless, contrast angiography remains the most common invasive method of vascular investigation for both diagnostic and therapeutic intervention. The angiogram usually provides the final information needed to decide whether or not to proceed with operation or endovascular interventions.
Digital subtraction angiography (DSA) offers some advantages over conventional cut-film angiography such as excellent visualization despite use of lower volumes of contrast media. In particular, when multilevel occlusive lesions limit the amount of contrast reaching distal vessels, supplemental use of digital subtraction angiographic techniques may enhance visualization and definition of anatomy. Intra-arterial DSA uses a portable, axially rotatable imaging device that can obtain views from different angles. DSA also allows for real-time video replay (Fig. 23-6). An entire extremity can be filmed with DSA using repeated injections of small amounts of contrast agent to obtain sequential angiographic images, the so-called pulse-chase technique.
Figure 23-6.
Digital subtraction angiography (DSA) provides excellent visualization of intravascular circulation with intra-arterial contrast administration. As depicted in this DSA study, multilevel lesions are demonstrated, which include a focal left iliac artery stenosis (large arrow), right superficial femoral occlusion (curved arrows), left superficial femoral stenosis (small arrow), and multiple tibial artery stenoses (arrowheads).
The most important and most controversial aspect of preoperative evaluation in patients with atherosclerotic disease requiring surgical intervention is the detection and subsequent management of associated coronary artery disease.7 Several studies have documented the existence of significant coronary artery disease in 40% to 50% or more of patients requiring peripheral vascular reconstructive procedures, 10% to 20% of whom may be relatively asymptomatic largely because of their inability to exercise.8 Myocardial infarction is responsible for the majority of both early and late postoperative deaths. Most available screening methods lack sensitivity and specificity to predict postoperative cardiac complications. There have been conflicting reports regarding the utility of preoperative dipyridamole-thallium nuclear imaging or dobutamine-echocardiography to stratify vascular patients in terms of perioperative cardiac morbidity and mortality. In nearly half of patients, thallium imaging proves to be unnecessary because cardiac risk can be predicted by clinical information alone.7 Even with coronary angiography, it is difficult to relate anatomic findings to functional significance and, hence, surgical risk. There are no data confirming that percutaneous coronary interventions or surgical revascularization prior to vascular surgical procedures impact mortality or incidence of myocardial infarctions. In fact, coronary angiography is associated with its own inherent risks, and patients undergoing coronary artery bypass grafting or coronary percutaneous transluminal angioplasty (PTA) before needed aortoiliac reconstructions are subjected to the risks and complications of both procedures.
The Coronary Artery Revascularization Prophylaxis (CARP) trial showed that coronary revascularization in patients with peripheral vascular disease and significant coronary artery disease, who are considered high risk for perioperative complications, did not reduce overall mortality or perioperative myocardial infarction.9 Additionally, patients who underwent prophylactic coronary revascularization had significant delays prior to undergoing their vascular procedure and increased limb morbidity compared to patients who did not. Studies do support improvement in cardiovascular and overall prognosis with medical optimization of patients. Therefore, use of perioperative β-blockade, as well as use of antiplatelet medication, statins, and angiotensin-converting enzyme inhibitors, is encouraged in vascular patients.10,11
BASIC PRINCIPLES OF ENDOVASCULAR THERAPY
Cardiovascular disease remains a major cause of mortality in the developed world since the beginning of the twenty-first century. Although surgical revascularization has played a predominant role in the management of patients with vascular disease, the modern treatment paradigms have evolved significantly with increased emphasis of catheter-based percutaneous interventions over the past two decades. The increasing role of this minimally invasive vascular intervention is fueled by various factors, including rapid advances in imaging technology, reduced morbidity and mortality in endovascular interventions, and faster convalescence following percutaneous therapy when compared to traditional operations. There is little doubt that with continued device development and refined image-guided technology, endovascular intervention will provide improved clinical outcomes and play an even greater role in the treatment of vascular disease.
The technique of percutaneous access for both the diagnostic and therapeutic management of vascular disease has resulted in tremendous changes in the practice of several subspecialties, including interventional radiology, invasive cardiology, and vascular surgery. The development of catheter and endoscopic instrumentation allows the vascular surgeon to operate via an intra- or extraluminal route. Endovascular techniques are now able to treat the full spectrum of vascular pathology, including stenoses and occlusions resulting from several etiologies, aneurysmal pathology, and traumatic lesions. Many of these procedures have only recently been developed and, as such, have not been investigated in a manner that would enable an accurate comparison with the more traditional methods of open surgical intervention. Long-term follow-up for these procedures is frequently lacking; however, because of the potential to treat patients with decreased mortality and morbidity, endovascular skills and techniques are being adopted into mainstream vascular surgery.
Needles are used to achieve percutaneous vascular access. The size of the needle will be dictated by the diameter of the guidewire used. Most often, an 18-gauge needle is used, as it will accept a 0.035-inch guidewire. A 21-gauge micropuncture needle will accept a 0.018-inch guidewire. The most popular access needle is the Seldinger needle, which can be used for single- and double-wall puncture techniques.
Femoral arterial puncture is the most common site for access. The common femoral artery (CFA) is punctured over the medial third of the femoral head, which is landmarked using fluoroscopy. The single-wall puncture technique requires a sharp, beveled needle tip and no central stylet. The anterior wall of the vessel is punctured with the bevel of the needle pointing up, and pulsatile back-bleeding indicates an intraluminal position. This method is most useful for graft punctures, patients with abnormal clotting profiles, or if thrombolytic therapy is anticipated. Once the needle assumes an intraluminal position, verified by pulsatile back-bleeding, the guidewire may be advanced. This is always passed gently and under fluoroscopic guidance to avoid subintimal dissection or plaque disruption. Double-wall puncture techniques are performed with a blunt needle that has a removable inner cannula. The introducer needle punctures both walls of the artery and is withdrawn until bleeding is obtained to confirm intraluminal position prior to advancing a guidewire. There can be troublesome bleeding from the posterior arterial wall puncture; therefore, single puncture techniques are preferred.
Retrograde femoral access is the most common arterial access technique (Fig. 23-7). The advantages of this technique include the size and fixed position of the CFA, as well as the relative ease of compression against the femoral head at the end of the procedure. Care should be taken to avoid puncturing the external iliac artery above the inguinal ligament because this can result in retroperitoneal hemorrhage secondary to ineffective compression of the puncture site. Likewise, puncturing too low, at or below the CFA bifurcation, can result in thrombosis or pseudoaneurysm formation of the superficial femoral artery (SFA) or profunda femoris artery (PFA). Antegrade femoral access is more difficult than retrograde femoral access, and there is a greater tendency to puncture the SFA, but it is invaluable when the aortic bifurcation cannot be traversed or when devices are not long enough to reach a lesion from a contralateral femoral access approach. Occasionally, when the distal aorta or bilateral iliac arteries are inaccessible because of the extent of atherosclerotic lesions, scarring, or presence of bypass conduits, the brachial artery must be used to obtain access for diagnostic and therapeutic interventions. The left brachial artery is punctured because this avoids the origin of the carotid artery and thus decreases the risk of catheter-related emboli to the brain. The artery is accessed with a micropuncture needle just proximal to the antecubital crease. The use of brachial access is associated with a higher risk of thrombosis and nerve injuries than femoral access.
Figure 23-7.
A. Antegrade femoral artery access. The needle is inserted just below the inguinal ligament in the common femoral artery whereby the guidewire is inserted in the ipsilateral superficial femoral artery. B. Brachial artery approach. The needle is inserted in a retrograde fashion in the brachial artery just above the antecubital fossa, whereby the guidewire is next inserted in the brachial artery.
Guidewires are used to introduce, position, and exchange catheters. A guidewire generally has a flexible and stiff end. In general, only the flexible end of the guidewire is placed in the vessel. All guidewires are composed of a stiff inner core and an outer tightly coiled spring that allows a catheter to track over the guidewire. There are five essential characteristics of guidewires: size, length, stiffness, coating, and tip configuration.
Guidewires come in different maximum transverse diameters, ranging from 0.011 to 0.038 inches. For most aortoiliac procedures, a 0.035-inch wire is most commonly used, whereas the smaller diameter 0.018-inch guidewires are reserved for selective small vessel angiography such as infrageniculate or carotid lesions. In addition to diameter size, guidewires come in varying lengths, usually ranging from 180 to 260 cm in length. Increasing the length of the wire always makes it more difficult to handle and increases the risk of contamination. While performing a procedure, it is important to maintain the guidewire across the lesion until the completion arteriogram has been satisfactorily completed.
The stiffness of the guidewire is also an important characteristic. Stiff wires allow for passage of large aortic stent graft devices without kinking. They are also useful when trying to perform sheath or catheter exchanges around a tortuous artery. An example of a stiff guidewire is the Amplatz wire. Hydrophilic coated guidewires, such as the Glidewire, have become invaluable tools for assisting in difficult catheterizations. The coating is primed by bathing the guidewire in saline solution. The slippery nature of this guidewire along with its torque capability significantly facilitate in difficult catheterizations. Guidewires also come in various tip configurations. Angled tip wires like the angled Glidewire can be steered to manipulate a catheter across a tight stenosis or to select a specific branch of a vessel. The Rosen wire has a soft curled end, which makes it ideal for renal artery stenting. The soft curl of this wire prevents it from perforating small renal branch vessels.
The hemostatic sheath is a device through which endovascular procedures are performed. The sheath acts to protect the vessel from injury as wires and catheters are introduced (Fig. 23-8). A one-way valve prevents bleeding through the sheath, and a side-port allows contrast or heparin flushes to be administered during the procedure. Sheaths are sized by their inner diameter. The most commonly used sheaths for percutaneous access have a 5- to 9-French inner diameter, but with open surgical exposure of the CFA, sheaths as large as 26 French can be introduced. Sheaths also vary in length, and long sheaths are available so that interventions remote from the site of arterial access can be performed.
A wide variety of catheters exist that differ primarily in the configuration of the tip. The multiple shapes permit access to vessels of varying dimensions and angulations. Catheters are used to perform angiography and protect the passage of balloons and stents, and can be used to direct the guidewire through tight stenoses or tortuous vessels.
Angioplasty balloons differ primarily in their length and diameter, as well as the length of the catheter shaft. As balloon technology has advanced, lower profiles have been manufactured (i.e., the size that the balloon assumes upon deflation). Balloons are used to perform angioplasty on vascular stenoses, to deploy stents, and to assist with additional expansion after insertion of self-expanding stents (Fig. 23-9). Besides length and diameter, operators need to be familiar with several other balloon characteristics. Noncompliant and low-compliance balloons tend to be inflated to their preset diameter and offer greater dilating force at the site of stenosis. Low-compliance balloons are the mainstay for peripheral intervention. Lower profile balloons are less likely to get caught during passage through stents and are easier to pull out of sheaths. Under fluoroscopic guidance, balloon inflation is performed until the waist of the atherosclerotic lesion disappears and the balloon is at the full profile. The duration of balloon inflation and pressures used for the angioplasty depend on the indication for the intervention and the location and characteristics of the lesion being treated. Frequently, several inflations are required to achieve a full profile of the balloon. Occasionally, a lower profile balloon is needed to predilate the tight stenosis so that the selected balloon catheter can cross the lesion. After inflation, most balloons do not regain their preinflation diameter and assume a larger profile. Trackability, pushability, and crossability of the balloon should all be considered when choosing a particular balloon. Lastly, shoulder length is an important characteristic to consider when selecting a balloon because of the potential to cause injury during performance of PTA in adjacent arterial segments. There is always risk of causing dissection or rupture during PTA; thus a completion angiogram is performed while the wire is still in place. Leaving the wire in place provides access for repeating the procedure, placing a stent or stent graft if warranted.
Vascular stents are commonly used after an inadequate angioplasty with dissection or elastic recoil of an arterial stenosis. They serve to buttress collapsible vessels and help prevent atherosclerotic restenosis. Appropriate indications for primary stenting of a lesion without an initial trial of angioplasty alone are evolving in manners that are dependent on the extent and site of the lesion. Stents are manufactured from a variety of metals including stainless steel, tantalum, cobalt-based alloy, and nitinol. Vascular stents are classified into two basic categories: balloon-expandable stents and self-expanding stents.
Self-expanding stents (Fig. 23-10) are deployed by retracting a restraining sheath and usually consist of Elgiloy (a cobalt, chromium, nickel alloy) or nitinol (a shape memory alloy composed of nickel and titanium), the latter of which will contract and assume a heat-treated shape above a transition temperature that depends on the composition of the alloy. Self-expanding stents will expand to a final diameter that is determined by stent geometry, hoop strength, and vessel size. The self-expanding stent is mounted on a central shaft and is placed inside an outer sheath. It relies on a mechanical spring-like action to achieve expansion. With deployment of these stents, there is some degree of foreshortening that has to be taken into account when choosing the area of deployment. In this way, self-expanding stents are more difficult to place with absolute precision. There are several advantages related to self-expanding stents. Self-expanding stents generally come in longer lengths than balloon-expandable stents and are therefore used to treat long and tortuous lesions. Their ability to continually expand after delivery allows them to accommodate adjacent vessels of different size. This makes these stents ideal for placement in the internal carotid artery. These stents are always oversized by 1 to 2 mm relative to the largest diameter of normal vessel adjacent to the lesion in order to prevent immediate migration.
Figure 23-10.
Self-expanding stents are made of tempered stainless steel or nitinol, an alloy of nickel and titanium, and are restrained when folded inside a delivery catheter. After being released from the restraining catheter, the self-expanding stents will expand to a final diameter that is determined by stent geometry, hoop strength, and vessel size.
Balloon-expandable stents are usually composed of stainless steel, mounted on an angioplasty balloon, and deployed by balloon inflation (Fig. 23-11). They can be manually placed on a chosen balloon catheter or obtained premounted on a balloon catheter. The capacity of a balloon-expandable stent to shorten in length during deployment depends on both stent geometry and the final diameter to which the balloon is expanded. These stents are more rigid and are associated with a shorter time to complete endothelialization. They are often of limited flexibility and have a higher degree of crush resistance when compared to self-expanding stents. This makes them ideal for short-segment lesions, especially those that involve the ostia such as proximal common iliac or renal artery stenosis.
Figure 23-11.
In a balloon-expandable stent, the stent is premounted on a balloon catheter. The balloon stretches the stent members beyond their elastic limit. The stent is deployed by full balloon expansion. This type of stent has a higher degree of crush resistance when compared to self-expanding stents, which is ideal for short-segment calcified ostial lesions.
The most exciting area of development in stents is the evolution of drug-eluting stents (DES). These stents are usually composed of nitinol and have various anti-inflammatory drugs bonded to them. Over time, the stents release the drug into the surrounding arterial wall and help prevent restenosis. Numerous randomized controlled trials have proven their benefit in coronary arteries.12 Clinical studies have similarly proved early efficacy of DES in the treatment of peripheral arterial disease.13,14
The combination of a metal stent covered with fabric gave birth to the first stent grafts. Covered stents have been designed with either a surrounding PTFE or polyester fabric and have been used predominantly for treatment of traumatic vascular lesions, including arterial disruption and arteriovenous fistulas (Fig. 23-12). However, these devices may well find a growing role in treatment of iliac or femoral arterial occlusive disease as well as popliteal aneurysms.
Endovascular aneurysm repair using the concept of stent grafts was initiated by Parodi in 1991.15 Since that time, a large number of endografts have been inserted under the auspice of clinical trials initially and now as Food and Drug Administration (FDA)–approved devices. Current available FDA-approved devices include the following: (a) AneuRx device (Medtronic/AVE, Santa Rosa, CA); (b) Gore Excluder device (WL Gore & Associates, Flagstaff, AZ); (c) Endologix Powerlink device (Endologix Inc., Irvine, CA); (d) Zenith device (Cook Inc., Bloomington, IN); (e) Talent device (Medtronic/AVE, Santa Rosa, CA); and (f) Endurant device (Medtronic/AVE, Santa Rosa, CA) for the treatment of abdominal aortic aneurysms. All of these devices require that patients have an infrarenal aneurysm with at least a 15-mm proximal aortic neck below the renal arteries and not greater than 60° of angulation. For those patients with associated common iliac artery aneurysmal disease, endovascular treatment can be achieved by initial coil embolization of the ipsilateral hypogastric artery with extension of the endovascular device into the external iliac artery. Newer generation endografts, including devices such as AFX Endovascular AAA System (Endologix Inc., Irvine, CA), Aorfix Flexible Stent (Lombard Medical Inc., Framingham, MA), and Ovation Prime Stent (TriVascular Inc., Santa Rosa, CA), are designed to overcome previous challenges of difficult anatomy by incorporating more flexible stents and lower profile delivery systems. Clinical trials are under way with devices that will expand indications to aneurysms involving the visceral segment of the abdominal aorta. The FDA has similarly approved several thoracic endograft devices for the treatment of descending thoracic aortic aneurysm. Early studies have demonstrated short-term efficacy of thoracic aortic devices in the treatment of traumatic aortic transections and aortic dissections.16,17,18 More experience with these devices exists in both Europe and Asia, and trials are under way in the United States with several devices.
CAROTID ARTERY DISEASE
Atherosclerotic occlusive plaque is by far the most common pathology seen in the carotid artery bifurcation. Thirty percent to 60% of all ischemic strokes are related to atherosclerotic carotid bifurcation occlusive disease. In the following section, we first focus our discussion on the clinical presentation, diagnosis, and management, including medical therapy, surgical carotid endarterectomy, and stenting, of atherosclerotic carotid occlusive disease. In the second part of the section, we provide a review on other less common nonatherosclerotic diseases involving the extracranial carotid artery, including kink and coil, fibromuscular dysplasia, arterial dissection, aneurysm, radiation arteritis, Takayasu’s arteritis, and carotid body tumor.
Approximately 700,000 Americans suffer a new or recurrent stroke each year.19 Eighty-five percent of all strokes are ischemic, and 15% are hemorrhagic. Hemorrhagic strokes are caused by head trauma or spontaneous disruption of intracerebral blood vessels. Ischemic strokes are due to hypoperfusion from arterial occlusion or, less commonly, to decreased flow resulting from proximal arterial stenosis and poor collateral network. Common causes of ischemic strokes are cardiogenic emboli in 35%, carotid artery disease in 30%, lacunar in 10%, miscellaneous in 10%, and idiopathic in 15%.19 The term cerebrovascular accident is often used interchangeably to refer to an ischemic stroke. A transient ischemic attack (TIA) is defined as a temporary focal cerebral or retinal hypoperfusion state that resolves spontaneously within 24 hours after its onset. However, the majority of TIAs resolve within minutes, and longer lasting neurologic deficits more likely represent a stroke. Recently, the term brain attack has been coined to refer to an acute stroke or TIA, denoting the condition as a medical emergency requiring immediate attention, similar to a heart attack.
Stroke due to carotid bifurcation occlusive disease is usually caused by atheroemboli (Fig. 23-13). The carotid bifurcation is an area of low flow velocity and low shear stress. As the blood circulates through the carotid bifurcation, there is separation of flow into the low-resistance internal carotid artery and the high-resistance external carotid artery. Characteristically, atherosclerotic plaque forms in the outer wall opposite to the flow divider (Fig. 23-14). Atherosclerotic plaque formation is complex, beginning with intimal injury, platelet deposition, smooth muscle cell proliferation, and fibroplasia, and leading to subsequent luminal narrowing. With increasing degree of stenosis in the internal carotid artery, flow becomes more turbulent, and the risk of atheroembolization escalates. The severity of stenosis is commonly divided into three categories according to the luminal diameter reduction: mild (<50%), moderate (50%–69%), and severe (70%–99%). Severe carotid stenosis is a strong predictor for stroke.20 In turn, a prior history of neurologic symptoms (TIA or stroke) is an important determinant for recurrent ipsilateral stroke. The risk factors for the development of carotid artery bifurcation disease are similar to those causing atherosclerotic occlusive disease in other vascular beds. Increasing age, male gender, hypertension, tobacco smoking, diabetes mellitus, homocysteinemia, and hyperlipidemia are well-known predisposing factors for the development of atherosclerotic occlusive disease.
Figure 23-13.
Stroke due to carotid bifurcation occlusive disease is usually caused by atheroemboli arising from the internal carotid artery, which provides the majority of blood flow to the cerebral hemisphere. With increasing degree of stenosis in the carotid artery, flow becomes more turbulent, and the risk of atheroembolization escalates.
Figure 23-14.
A. The carotid bifurcation is an area of low flow velocity and low shear stress. As the blood circulates through the carotid bifurcation, there is separation of flow into the low-resistance internal carotid artery and the high-resistance external carotid artery. B. The carotid atherosclerotic plaque typically forms in the outer wall opposite to the flow divider due in part to the effect of the low shear stress region, which also creates a transient reversal of flow during the cardiac cycle.
TIA is a focal loss of neurologic function, lasting for less than 24 hours. Crescendo TIAs refer to a syndrome comprising repeated TIAs within a short period of time that is characterized by complete neurologic recovery in between. At a minimum, the term should probably be reserved for those with either daily events or multiple resolving attacks within 24 hours. Hemodynamic TIAs represent focal cerebral events that are aggravated by exercise or hemodynamic stress and typically occur after short bursts of physical activity, postprandially, or after getting out of a hot bath. It is implied that these are due to severe extracranial disease and poor intracranial collateral recruitment. Reversible ischemic neurologic deficits refer to ischemic focal neurologic symptoms lasting longer than 24 hours but resolving within 3 weeks. When a neurologic deficit lasts longer than 3 weeks, it is considered a completed stroke. Stroke in evolution refers to progressive worsening of the neurologic deficit, either linearly over a 24-hour period or interspersed with transient periods of stabilization and/or partial clinical improvement.
Patients who suffer cerebrovascular accidents typically present with three categories of symptoms including ocular symptoms, sensory/motor deficit, and/or higher cortical dysfunction. The common ocular symptoms associated with extracranial carotid artery occlusive disease include amaurosis fugax and presence of Hollenhorst plaques. Amaurosis fugax, commonly referred to as transient monocular blindness, is a temporary loss of vision in one eye that patients typically describe as a window shutter coming down or grey shedding of the vision. This partial blindness usually lasts for a few minutes and then resolves. Most of these phenomena (>90%) are due to embolic occlusion of the main artery or the upper or lower divisions. Monocular blindness progressing over a 20-minute period suggests a migrainous etiology. Occasionally, the patient will recall no visual symptoms while the optician notes a yellowish plaque within the retinal vessels, which is also known as Hollenhorst plaque. These plaques are frequently derived from cholesterol embolization from the carotid bifurcation and warrant further investigation. Additionally, several ocular symptoms may be caused by microembolization from extracranial carotid diseases including monocular visual loss due to retinal artery or optic nerve ischemia, the ocular ischemia syndrome, and visual field deficits secondary to cortical infarction and ischemia of the optic tracts. Typical motor and/or sensory symptoms associated with cerebrovascular accidents are lateralized or focal neurologic deficits. Ischemic events tend to have an abrupt onset, with the severity of the insult being apparent from the onset and not usually associated with seizures or paresthesia. In contrast, they represent loss or diminution of neurologic function. Furthermore, motor or sensory deficits can be unilateral or bilateral, with the upper and lower limbs being variably affected depending on the site of the cerebral lesion. The combination of a motor and sensory deficit in the same body territory is suggestive of a cortical thromboembolic event as opposed to lacunar lesions secondary to small vessel disease of the penetrating arterioles. However, a small proportion of the latter may present with a sensorimotor stroke secondary to small vessel occlusion within the posterior limb of the internal capsule. Pure sensory and pure motor strokes and those strokes where the weakness affects one limb only or does not involve the face are more typically seen with lacunar as opposed to cortical infarction. A number of higher cortical functions, including speech and language disturbances, can be affected by thromboembolic phenomena from the carotid artery, with the most important clinical example for the dominant hemisphere being dysphasia or aphasia and visuospatial neglect being an example of nondominant hemisphere injury.
Duplex ultrasonography is the most widely used screening tool to evaluate for atherosclerotic plaque and stenosis of the extracranial carotid artery. It is also commonly used to monitor patients serially for progression of disease or after intervention (carotid endarterectomy or angioplasty). Duplex ultrasound of the carotid artery combines B-mode gray scale imaging and Doppler waveform analysis. Characterization of the carotid plaque on gray scale imaging provides useful information about its composition. However, there are currently no universal recommendations that can be made based solely on the sonographic appearance of the plaque. On the other hand, criteria have been developed and well refined for grading the degree of carotid stenosis based primarily on Doppler-derived velocity waveforms.
The external carotid artery has a high-resistance flow pattern with a sharp systolic peak and a small amount of flow in diastole. In contrast, a normal internal carotid artery will have a low-resistance flow pattern with a broad systolic peak and a large amount of flow during diastole. The flow pattern in the common carotid artery resembles that in the internal carotid artery, as 80% of the flow is directed to the internal carotid artery, with waveforms that have broad systolic peaks and moderate amount of flow during diastole. Conventionally, velocity measurements are recorded in the common, external, carotid bulb, and the proximal, mid, and distal portions of the internal carotid artery. Characteristically, the peak systolic velocity is increased at the site of the vessel stenosis. The end-diastolic velocity is increased with greater degree of stenosis. In addition, stenosis of the internal carotid artery can lead to color shifts with color mosaics indicating a poststenotic turbulence. Dampening of the Doppler velocity waveforms is typically seen in areas distal to severe carotid stenosis where blood flow is reduced. It is well known that occlusion of the ipsilateral internal carotid artery can lead to a “falsely” elevated velocity on the contralateral side due to an increase in compensatory blood flow. In the presence of a high-grade stenosis or occlusion of the internal carotid artery, the ipsilateral common carotid artery displays high flow resistance waveforms, similar to those seen in the external carotid artery. If there is a significant stenosis in the proximal common carotid artery, its waveforms may be dampened with low velocities.
The Doppler grading systems of carotid stenosis were initially established by comparison to angiographic findings of disease. Studies have shown variability in the measurements of the duplex properties by different laboratories, as well as heterogeneity in the patient population, study design, and techniques. One the most commonly used classifications was established at the University of Washington School of Medicine in Seattle. Diameter reduction of 50% to 79% is defined by peak systolic velocity greater than 125 cm/s with extensive spectral broadening. For stenosis in the range of 80% to 99%, the peak systolic velocity is greater than 125 cm/s, and peak diastolic velocity is greater than 140 cm/s. The ratio of internal carotid to common carotid artery peak systolic velocity has also been part of various ultrasound diagnostic classifications. A ratio greater than 4 is a great predictor of angiographic stenosis of 70% to 99%. A multispecialty consensus panel has developed a set of criteria for grading carotid stenosis by duplex examination (Table 23-3).21
DEGREE OF STENOSIS (%) | ICA PSV (CM/S) | ICA/CCA PSV RATIO | ICA EDV (CM/S) | PLAQUE ESTIMATE (%)a |
---|---|---|---|---|
Normal | <125 | <2.0 | <40 | None |
<50 | <125 | <2.0 | <40 | <50 |
50–69 | 125–230 | 2.0–4.0 | 40–100 | ≥50 |
≥70 to less than near occlusion | >230 | >4.0 | >100 | ≥50 |
Near occlusion | High, low, or not detected | Variable | Variable | Visible |
Total occlusion | Not detected | Not applicable | Not detected | Visible, no lumen |
MRA is increasingly being used to evaluate for atherosclerotic carotid occlusive disease and intracranial circulation. MRA is noninvasive and does not require iodinated contrast agents. MRA uses phase contrast or time-of-flight, with either two-dimensional or three-dimensional data sets for greater accuracy. Three-dimensional contrast-enhanced MRA allows data to be obtained in coronal and sagittal planes with improved image qualities due to shorter study time. In addition, the new MRA techniques allow for better reformation of images in various planes to allow better grading of stenosis. There have been numerous studies comparing the sensitivity and specificity of MRA imaging for carotid disease to duplex and selective contrast angiography.22 Magnetic resonance imaging (MRI) of the brain is essential in the assessment of acute stroke patients. MRI with diffusion-weighted imaging can differentiate areas of acute ischemia, areas still at risk for ischemia (penumbra), and chronic cerebral ischemic changes. However, computed tomography (CT) imaging remains the most expeditious test in the evaluation of acute stroke patients to rule out intracerebral hemorrhage. Recently, multidetector CTA has gained increasing popularity in the evaluation of carotid disease.23 This imaging modality can provide volume rendering, which allows rotation of the object with accurate anatomic structures from all angles (Fig. 23-15). The advantages of CTA over MRA include faster data acquisition time and better spatial resolution. However, grading of carotid stenosis by CTA requires further validation at the time of this writing before it can be widely applied.
Figure 23-15.
A. Carotid computed tomography angiography is a valuable imaging modality that can provide a three-dimensional image reconstruction with high image resolution. A carotid artery occlusion is noted in the internal carotid artery B. The entire segment of extracranial carotid artery is visualized from the thoracic compartment to the base of skull.
Historically, DSA has been the gold standard test to evaluate the extra- and intracranial circulation (Fig. 23-16). This is an invasive procedure, typically performed via a transfemoral puncture, and involves selective imaging of the carotid and vertebral arteries using iodinated contrast. The risk of stroke during cerebral angiography is generally reported at approximately 1% and is typically due to atheroembolization related to wire and catheter manipulation in the arch aorta or proximal branch vessels. Over the last few decades, however, the incidence of neurologic complications following angiography has been reduced, due to the use of improved guidewires and catheters, better resolution digital imaging, and increased experience. Local access complications of angiography are infrequent and include development of hematoma, pseudoaneurysm, distal embolization, and acute vessel thrombosis. Currently, selective angiography is particularly used for patients with suspected intracranial disease and for patients in whom percutaneous revascularization is considered. The techniques of carotid angioplasty and stenting for carotid bifurcation occlusive disease are described in detail later in this chapter. We generally use CTA or MRA to get information about the aortic arch anatomy and presence of concomitant intracranial disease and collateral pathway in planning our strategy for carotid stenting or endarterectomy.
Conventionally, patients with carotid bifurcation occlusive disease are divided into two broad categories: patients without prior history of ipsilateral stroke or TIA (asymptomatic) and those with prior or current ipsilateral neurologic symptoms (symptomatic). It is estimated that 15% of all strokes are preceded by a TIA. The 90-day risk of a stroke in a patient presenting with a TIA is 3% to 17%.19 According to the Cardiovascular Health Study, a longitudinal population-based study of coronary artery disease and stroke in men and women, the prevalence of TIA in men was 2.7% for ages of 65 and 69 and 3.6% for ages 75 to 79; the prevalence in women was 1.4% and 4.1%, respectively.24 There have been several studies reporting on the effectiveness of stroke prevention with medical treatment and carotid endarterectomy for symptomatic patients with moderate to severe carotid stenosis. Early and chronic aspirin therapy has been shown to reduce stroke recurrence rate in several large clinical trials.25
Currently, most stroke neurologists prescribe both aspirin and clopidogrel for secondary stroke prevention in patients who have experienced a TIA or stroke.19 In patients with symptomatic carotid stenosis, the degree of stenosis appears to be the most important predictor in determining risk for an ipsilateral stroke. The risk of a recurrent ipsilateral stroke in patients with severe carotid stenosis approaches 40%. Two large multicenter randomized clinical trials, the European Carotid Surgery Trial (ECST) and the North American Symptomatic Carotid Endarterectomy Trial (NASCET), have both shown a significant risk reduction in stroke for patients with symptomatic high-grade stenosis (70%–99%) undergoing carotid endarterectomy when compared to medical therapy alone.26,27 There has been much discussion regarding the different methodology used in the measurement of carotid stenosis and calculation of the life-table data between the two studies, yet they both studies had similar results.28 Findings of these two landmark trials have also been reanalyzed in many subsequent publications. The main conclusions of the trials remain validated and widely acknowledged. Briefly, the NASCET study showed that for high-grade carotid stenosis, the cumulative risk of ipsilateral stroke was 26% in the medically treated group and 9% in the surgically treated group at 2 years. For patients with moderate carotid artery stenosis (50%–69%), the benefit of carotid endarterectomy is less but still favorable when compared to medical treatment alone; the 5-year fatal or nonfatal ipsilateral stroke rate was 16% in the surgically treated group versus 22% in the medically treated group.29 The risk of stroke was similar for the remaining group of symptomatic patients with less than 50% carotid stenosis, whether they had endarterectomy or medical treatment alone. The ECST reported similar stroke risk reduction for patients with severe symptomatic carotid stenosis and no benefit in patients with mild stenosis, when carotid endarterectomy was performed versus medical therapy.27
The optimal timing of carotid intervention after acute stroke, however, remains debatable. Earlier studies showed an increased rate of postoperative stroke exacerbation and conversion of a bland to hemorrhagic infarction when carotid endarterectomy was carried out within 5 to 6 weeks after acute stroke. The dismal outcome reported in the early experience was likely related to poor patient selection. The rate of stroke recurrence is not insignificant during the interval period and may be reduced with early intervention for symptomatic carotid stenosis. Contemporary series have demonstrated acceptable low rates of perioperative complications in patients undergoing carotid endarterectomy within 4 weeks after acute stroke.29 In a recent retrospective series, carotid artery stenting when performed early (<2 weeks) after the acute stroke was associated with higher mortality than when delayed (>2 weeks).30
Whereas there is universal agreement that carotid revascularization (endarterectomy or stenting) is effective in secondary stroke prevention for patients with symptomatic moderate and severe carotid stenosis, the management of asymptomatic patients remains an important controversy to be resolved. Generally, the detection of carotid stenosis in asymptomatic patients is related to the presence of a cervical bruit or based on screening duplex ultrasound findings. In one of the earlier observational studies, the authors showed that the annual occurrence rate of neurologic symptoms was 4% in a cohort of 167 patients with asymptomatic cervical bruits followed prospectively by serial carotid duplex scan.31 The mean annual rate of carotid stenosis progression to a greater than 50% stenosis was 8%. The presence of or progression to a greater than 80% stenosis correlated highly with either the development of a total occlusion of the internal carotid artery or new symptoms. The major risk factors associated with disease progression were cigarette smoking, diabetes mellitus, and age. This study supported the contention that it is prudent to follow a conservative course in the management of asymptomatic patients presenting with a cervical bruit.
One of the first randomized clinical trials on the treatment of asymptomatic carotid artery stenosis was the Asymptomatic Carotid Atherosclerosis Study (ACAS), which evaluated the benefits of medical management with antiplatelet therapy versus carotid endarterectomy.32 Over a 5-year period, the risk of ipsilateral stroke in individuals with a carotid artery stenosis greater than 60% was 5.1% in the surgical arm. On the other hand, the risk of ipsilateral stroke in patients treated with medical management was 11%. Carotid endarterectomy produced a relative risk reduction of 53% over medical management alone. The results of a larger randomized trial from Europe, the Asymptomatic Carotid Surgery Trial (ACST), recently confirmed similar beneficial stroke risk reduction for patients with asymptomatic, greater than 70% carotid stenosis undergoing endarterectomy versus medical therapy.33 An important point derived from this latter trial was that even with improved medical therapy, including the addition of statin drugs and clopidogrel, medical therapy was still inferior to endarterectomy in the primary stroke prevention for patients with high-grade carotid artery stenosis. It is generally agreed that asymptomatic patients with severe carotid stenosis (80%–99%) are at significantly increased risk for stroke and stand to benefit from either surgical or endovascular revascularization. However, revascularization for asymptomatic patients with a less severe degree of stenosis (60%–79%) remains controversial.
Currently, the argument is no longer whether medical therapy alone is inferior to surgical endarterectomy in stroke prevention for severe carotid stenosis. Rather, the debate now revolves around whether carotid angioplasty and stenting produce the same benefits demonstrated by carotid endarterectomy. Since carotid artery stenting was approved by the FDA for clinical application in 2004, this percutaneous procedure has become a treatment alternative in patients who are deemed “high risk” for endarterectomy (Table 23-4). In contrast to many endovascular peripheral arterial interventions, percutaneous carotid stenting represents a much more challenging procedure, because it requires complex catheter-based skills using the 0.014-inch guidewire system and distal protection device. Moreover, current carotid stent devices predominantly use the monorail guidewire system, which requires more technical agility compared with the over-the-wire catheter system that is routinely used in peripheral interventions. This percutaneous intervention often requires balloon angioplasty and stent placement through a long carotid guiding sheath via a groin approach. Poor technical skills can result in devastating treatment complications such as stroke, which can occur in part due to plaque embolization during the balloon angioplasty and stenting of the carotid artery. Because of these various procedural components that require high technical proficiency, many early clinical investigations of carotid artery stenting, which included physicians with little or no carotid stenting experience, resulted in alarmingly poor clinical outcomes. A recent Cochrane review noted that, before 2006, a total of 1269 patients had been studied in five randomized controlled trials comparing percutaneous carotid intervention and surgical carotid reconstruction.34 Taken together, these trials revealed that carotid artery stenting had a greater procedural risk of stroke and death when compared to carotid endarterectomy (odds ratio, 1.33; 95% confidence interval, 0.86 to 2.04). Additionally, a greater incidence of carotid restenosis was noted in the stenting group than the endarterectomy cohorts.
ANATOMIC FACTORS | PHYSIOLOGIC FACTORS |
---|---|
• High carotid bifurcation (above C2 vertebral body) | • Age ≥80 years • Left ventricular ejection fraction ≤30% |
• Low common carotid artery (below clavicle) • Contralateral carotid occlusion • Restenosis of ipsilateral prior carotid endarterectomy • Previous neck irradiation | • New York Heart Association class III/IV congestive heart failure • Unstable angina: Canadian Cardiovascular Society class III/IV angina pectoris • Recent myocardial infarction |
• Prior radical neck dissection • Contralateral laryngeal nerve palsy • Presence of tracheostomy | • Clinically significant cardiac disease (congestive heart failure, abnormal stress test, or need for coronary revascularization) • Severe chronic obstructive pulmonary disease • End-stage renal disease on dialysis |
However, the constant improvement of endovascular devices, procedural techniques, and adjunctive pharmacologic therapy will likely improve the treatment success of percutaneous carotid intervention. Critical appraisals of several prospective randomized trials comparing the efficacy of carotid stenting versus endarterectomy are available for review.35 Two recently published randomized controlled trial, the Carotid Revascularization Endarterectomy Versus Stent Trial (CREST) and the International Carotid Stenting Study (ICSS) have reported somewhat differing results.36 CREST compared the efficacy of carotid endarterectomy and carotid stenting in both symptomatic and asymptomatic patients.37 Primary end points included 30-day periprocedural composite death, stroke, myocardial infarction, or any ipsilateral stroke up to 4 years. CREST investigators reported no difference between stenting (5.2%) and endarterectomy (4.5%) in terms of primary end point. When each variable was independently analyzed, there was a higher rate of stroke in the stenting group at 30 days (4.1% vs. 2.3%) and a higher rate of myocardial infarction in the endarterectomy group (2.3% vs. 1.1%). The ICSS was a multicenter, international, randomized controlled trial comparing carotid stenting versus endarterectomy in patients with symptomatic carotid stenosis.38 The risk of stroke, death, and myocardial infarction in the stenting group (8.5%) was significantly higher than in the surgical arm (5.2%). The finding that carotid endarterectomy is safer than carotid stenting is also supported by the results of an MRI substudy, which showed significantly more new lesions by diffusion-weighted imaging in the carotid stenting than the carotid endarterectomy patients.
All available randomized studies have provided some answers and raised some questions. Some ongoing clinical trials will undoubtedly provide more insights on the efficacy of carotid stenting in the near future. Currently, the Society for Vascular Surgeons recommends carotid endarterectomy as first-line treatment for most symptomatic patients with stenosis of 50% to 99% and asymptomatic patients with stenosis of 60% to 99%.39 The perioperative risk of stroke and death in asymptomatic patients must be below 3% to ensure benefit for the patient. Carotid artery stenting should be reserved for symptomatic patients with stenosis of 50% to 99% at high risk for carotid endarterectomy for anatomic or medical reasons. Carotid artery stenting is not recommended for asymptomatic patients at this time. Asymptomatic patients at high risk for intervention or with a life expectancy of less than 3 years should be considered for medical management as the first-line therapy.
Although carotid endarterectomy is one of the earliest vascular operations ever described and its techniques have been perfected in the last two decades, surgeons continue to debate many aspects of this procedure. For instance, there is no universal agreement with regard to the best anesthetic of choice, the best intraoperative cerebral monitoring, whether to “routinely” shunt, open versus eversion endarterectomy, and patch versus primary closure. Various anesthetic options are available for patient undergoing carotid endarterectomy including general, local, and regional anesthesia. Typically the anesthesia of choice depends on the preference of the surgeon, anesthesiologist, and patient. However, depending on the anesthetic given, the surgeon must decide whether intraoperative cerebral monitoring is necessary or intra-arterial carotid shunting will be used. In general, if the patient is awake, then his or her abilities to respond to commands during carotid clamp period determine the adequacy of collateral flow to the ipsilateral hemisphere. On the other hand, intraoperative electroencephalogram (EEG) or transcranial power Doppler (TCD) has been used to monitor for adequacy of cerebral perfusion during the clamp period for patients undergoing surgery under general anesthesia. Focal ipsilateral decreases in amplitudes and slowing of EEG waves are indicative of cerebral ischemia. Similarly, a decrease to less than 50% of baseline velocity in the ipsilateral middle cerebral artery is a sign of cerebral ischemia. For patients with poor collateral flow exhibiting signs of cerebral ischemia, intra-arterial carotid shunting with removal of the clamp will restore cerebral flow for the remaining part of the surgery. Stump pressures have been used to determine the need for intra-arterial carotid shunting. Some surgeons prefer to shunt all patients on a routine basis and do not use intraoperative cerebral monitoring.
The patient’s neck is slightly hyperextended and turned to the contralateral side, with a roll placed between the shoulder blades. An oblique incision is made along the anterior border of the sternocleidomastoid muscle centered on top of the carotid bifurcation (Fig. 23-17). The platysma is divided completely. Typically tributaries of the anterior jugular vein are ligated and divided. The dissection is carried medial to the sternocleidomastoid. The superior belly of the omohyoid muscle is usually encountered just anterior to the common carotid artery. This muscle can be divided. The carotid fascia is incised, and the common carotid artery is exposed. The common carotid artery is mobilized cephalad toward the bifurcation. The dissection of the carotid bifurcation can cause reactive bradycardia related to stimulation of the carotid body. This reflex can be blunted with injection of lidocaine 1% into the carotid body or reversed with administration of intravenous atropine. A useful landmark in the dissection of the carotid bifurcation is the common facial vein. This vein can be ligated and divided. Frequently the 12th cranial nerve (hypoglossal nerve) traverses the carotid bifurcation just behind the common facial vein. The external carotid artery is mobilized just enough to get a clamp across. Often, a branch of the external carotid artery crossing to the sternocleidomastoid can be divided to allow further cephalad mobilization of the internal carotid artery. For high bifurcation, division of the posterior belly of the digastric muscle is helpful in establishing distal exposure of the internal carotid artery.
Intravenous heparin sulfate (1 mg/kg) is routinely administered just prior to carotid clamping. The internal carotid artery is clamped first using a soft noncrushing vascular clamp to prevent distal embolization. The external and common carotid arteries are clamped subsequently. A longitudinal arteriotomy is made in the distal common carotid artery and extended into the bulb and past the occlusive plaque into the normal part of the internal carotid artery. Endarterectomy is carried out to remove the occlusive plaque (Fig. 23-18). If necessary, a temporary shunt can be inserted from the common carotid artery to the internal carotid artery to maintain continuous antegrade cerebral blood flow (Fig. 23-19). Typically, a plane is teased out from the vessel wall, and the entire plaque is elevated and removed. The distal transition line in the internal carotid artery where the plaque had been removed must be examined carefully and should be smooth. Tacking sutures are placed when an intimal flap remains in this transition to ensure no obstruction to flow (Fig. 23-20). The occlusive plaque is usually removed from the origin of the external carotid artery using the eversion technique. The endarterectomized surface is then irrigated and any debris removed. A patch (autogenous saphenous vein, synthetic such as polyester, PTFE, or biologic material) is sewn to close the arteriotomy (Fig. 23-21). Whether patch closure is necessary in all patients and which patch is the best remain controversial. However, most surgeons agree that patch closure is indicated particularly for the small vessel (<7 mm). The eversion technique has also been advocated for removing the plaque from the internal carotid artery. In the eversion technique, the internal carotid artery is transected at the bulb, the edges of the divided vessel are everted, and the occluding plaque is “peeled” off the vessel wall. The purported advantages of the eversion technique are no need for patch closure and a clear visualization of the distal transition area. Reported series have not shown a clear superiority of one technique over the others.40 Surgeons will likely continue to use the technique of their choice. Just prior to completion of the anastomosis to close the arteriotomy, we thoroughly flush the vessels of any potential debris. When the arteriotomy is closed, flow is restored to the external carotid artery first and to the internal carotid artery second. Intravenous protamine sulfate can be given to reverse the effect of heparin anticoagulation following carotid endarterectomy. The wound is closed in layers. After surgery, the patient’s neurologic condition is asserted in the operating room prior to transfer to the recovery area.
Figure 23-18.
A. During carotid endarterectomy, vascular clamps are applied in the common carotid, external carotid, and internal carotid arteries. Carotid plaque is elevated from the carotid lumen. B. Carotid plaque is removed, and the arteriotomy is closed either primarily or with a patch angioplasty.
Most patients tolerate carotid endarterectomy very well and typically are discharged home within 24 hours after surgery. Complications after endarterectomy are infrequent but can be potentially life-threatening or disabling. Acute ipsilateral stroke is a dreaded complication following carotid endarterectomy. Cerebral ischemia can be due to either intraoperative or postoperative events. Embolizations from the occlusive plaque or prolonged cerebral ischemia are potential causes of intraoperative stroke. The most common cause of postoperative stroke is due to embolization. Less frequently, acute carotid artery occlusion can cause acute postoperative stroke. This is usually due to carotid artery thrombosis related to closure of the arteriotomy, an occluding intimal flap, or distal carotid dissection. When patients experience acute symptoms of neurologic ischemia after endarterectomy, immediate intervention may be indicated. Carotid duplex scan can be done expeditiously to assess patency of the extracranial internal carotid artery. Re-exploration is mandated for acute carotid artery occlusion. Cerebral angiography can be useful if intracranial revascularization is considered.
Local complications related to surgery include excessive bleeding and cranial nerve palsies. Postoperative hematoma in the neck after carotid endarterectomy can lead to devastating airway compromise. Any expanding hematoma should be evacuated and active bleeding stopped. Securing an airway is critical and can be extremely difficult in patients with large postoperative neck hematoma. The reported incidence of postoperative cranial nerve palsies after carotid endarterectomy varies from 1% to 30%.41 Well-recognized injuries involve the marginal mandibular, vagus, hypoglossal, superior laryngeal, and recurrent laryngeal nerves. Often these are traction injuries but can also be due to severance of the respective nerves.
Percutaneous carotid artery stenting has become an accepted alternative treatment in the management of patients with carotid bifurcation disease (Fig. 23-22). The perceived advantages of percutaneous carotid revascularization are related to the minimal invasiveness of the procedure compared to surgery. There are anatomic conditions based on angiographic evaluation in which carotid artery stenting should be avoided due to increased procedure-related risks (Table 23-5). In preparation for carotid stenting, the patient should be given oral clopidogrel 3 days prior to the intervention if the patient was not already taking the drug. The procedure is done in either the operating room with angiographic capabilities or in a dedicated angiography room. The patient is placed in the supine position. The patient’s blood pressure and cardiac rhythm are closely monitored.
• Extensive carotid calcification • Polypoid or globular carotid lesions • Severe tortuosity of the common carotid artery • Long-segment stenoses (>2 cm in length) • Carotid artery occlusion • Severe intraluminal thrombus (angiographic defects) • Extensive middle cerebral artery atherosclerosis |
To gain access to the carotid artery, a retrograde transfemoral approach is most commonly used as the access site for carotid intervention. Using the Seldinger technique, we insert a diagnostic 5- or 6-French sheath in the CFA. A diagnostic arch aortogram is obtained. The carotid artery to be treated is then selected using a 5-French diagnostic catheter, and contrast is injected to show the carotid anatomy. It is important to assess the contralateral carotid artery, vertebrobasilar, and intracranial circulation if these are not known based on the preoperative noninvasive studies. Once the decision is made to proceed with carotid artery stenting, with the tip of the diagnostic catheter still in the common carotid artery, a 0.035-inch, 260-cm long stiff guidewire is placed in the ipsilateral external carotid artery. Anticoagulation with intravenous bivalirudin bolus (0.75 mg/kg) followed by an infusion rate of 2.5 mg/kg/h for the remainder of the procedure is routinely administered. Next the diagnostic catheter is withdrawn and a 90-cm, 6-French guiding sheath is advanced into the common carotid artery over the stiff glide wire. It is critical not to advance the sheath beyond the occlusive plaque in the carotid bulb. The stiff wire is then removed, and preparation is made to deploy the distal embolic protection device (EPD). Several distal EPDs are available (Table 23-6). The EPD device is carefully deployed beyond the target lesion. With regard to the carotid stents, there are several stents that have received approval from the FDA and are commercially available for carotid revascularization (Table 23-7). All current carotid stents use the rapid-exchange monorail 0.014-inch platform. The size selection is typically based on the size of common carotid artery. Predilatation using a 4-mm balloon may be necessary to allow passage of the stent delivery catheter. Once the stent is deployed across the occlusive plaque, postdilatation is usually performed using a ≤5.5-mm balloon. It is noteworthy that balloon dilation of the carotid bulb may lead to immediate bradycardia due to stimulation of the glossopharyngeal nerve. The EPD is then retrieved and the procedure is completed with removal of the sheath from the femoral artery. The puncture site is closed using available closure device or with manual compression. Throughout the procedure, the patient’s neurologic function is closely monitored. The bivalirudin infusion is stopped and the patient is kept on clopidogrel (75 mg daily) for at least 1 month and aspirin indefinitely.
MECHANISM | NAME OF EPD | PORE SIZE (μM) |
---|---|---|
Distal balloon occlusion | PercuSurge Guard Wire, Export catheter (Medtronic) | NA |
Distal filter | Angioguard (Cordis) Accunet (Abbott) Emboshield (Abbott) FilterWire (Boston Scientific) SpiderRx (EV3) | 100 150 140 110 <100 |
Flow reversala | Parodi Neuro Protection (Gore) | NA |
NAME OF STENT | MANUFACTURER | CELL DESIGN | TAPERED STENT | DELIVERY SYSTEM SIZE (FRENCH) |
---|---|---|---|---|
Acculink | Abbott | Open | Yes | 6 |
Exact | Abbott | Closed | Yes | 6 |
NexStent | Boston Scientific | Closed | Self-tapering | 5 |
Protégé RX | EV3 | Open | Yes | 6 |
Precise RX | Cordis | Open | No | 6 |
Exponent | Medtronic | Open | No | 6 |
Although there have been no randomized trials comparing carotid stenting with and without EPD, the availability of EPDs appears to have reduced the risk of distal embolization and stroke. The results of the various clinical trials and registries of carotid stenting have been reported and compared. It is well known that distal embolization as detected by TCD is much more frequent with carotid stenting even with EPD, when compared with carotid endarterectomy. However, the clinical significance of the distal embolization detected by TCD is not clear because most are asymptomatic. Acute carotid stent thrombosis is rare. The incidence of in-stent carotid restenosis is not well known but is estimated at 10% to 30%. Duplex surveillance shows elevated peak systolic velocities within the stent after carotid stenting not infrequently. However, velocity criteria are being formulated to determine the severity of in-stent restenosis after carotid stenting by ultrasound duplex.42 It appears that systolic velocities exceeding 300 to 400 cm/s would represent >70% to 80% restenosis. Bradycardia and hypotension occur in up to 20% of patients undergoing carotid stenting.43 Systemic administration of atropine is usually effective in reversing the bradycardia. Other technical complications of carotid stenting are infrequent and include carotid artery dissection and access site complications, such as groin hematoma, femoral artery pseudoaneurysm, distal embolization, and acute femoral artery thrombosis.
A carotid coil consists of an excessive elongation of the internal carotid artery producing tortuosity of the vessel (Fig. 23-23). Embryologically, the carotid artery is derived from the third aortic arch and dorsal aortic root and is uncoiled as the heart and great vessels descend into the mediastinum. In children, carotid coils appear to be congenital in origin. In contrast, elongation and kinking of the carotid artery in adults are associated with the loss of elasticity and an abrupt angulation of the vessel. Kinking is more common in women than men. Cerebral ischemic symptoms caused by kinks of the carotid artery are similar to those from atherosclerotic carotid lesions but are more likely due to due to cerebral hypoperfusion than embolic episodes. Classically, sudden head rotation, flexion, or extension can accentuate the kink and provoke ischemic symptoms. Most carotid kinks and coils are found incidentally on carotid duplex scan. However, interpretation of the Doppler frequency shifts and spectral analysis in tortuous carotid arteries can be difficult because of the uncertain angle of insonation. Cerebral angiography, with multiple views taken in neck flexion, extension, and rotation, is useful in the determination of the clinical significance of kinks and coils.
Fibromuscular dysplasia (FMD) usually involves medium-sized arteries that are long and have few branches (Fig. 23-24). Women in the fourth or fifth decade of life are more commonly affected than men. Hormonal effects on the vessel wall are thought to play a role in the pathogenesis of FMD. FMD of the carotid artery is commonly bilateral, and in about 20% of patients, the vertebral artery is also involved.44 An intracranial saccular aneurysm of the carotid siphon or middle cerebral artery can be identified in up to 50% of the patients with FMD. Four histologic types of FMD have been described in the literature. The most common type is medial fibroplasia, which may present as a focal stenosis or multiple lesions with intervening aneurysmal outpouchings. The disease involves the media with the smooth muscle being replaced by fibrous connective tissue. Commonly, mural dilations and microaneurysms can be seen with this type of FMD. Medial hyperplasia is a rare type of FMD, with the media demonstrating excessive amounts of smooth muscle. Intimal fibroplasia accounts for 5% of all cases and occurs equally in both sexes. The media and adventitia remain normal, and there is accumulation of subendothelial mesenchymal cells with a loose matrix of connective tissue causing a focal stenosis in adults. Finally, premedial dysplasia represents a type of FMD with elastic tissue accumulating between the media and adventitia. FMD can also involve the renal and external iliac arteries. It is estimated that approximately 40% of patients with FMD present with a TIA due to embolization of platelet aggregates.44 DSA demonstrates the characteristic “string of beads” pattern, which represents alternating segments of stenosis and dilatation. The string of beads can also be shown noninvasively by CTA or MRA. FMD should be suspected when an increased velocity is detected across a stenotic segment without associated atherosclerotic changes on carotid duplex ultrasound. Antiplatelet medication is the generally accepted therapy for asymptomatic lesions. Endovascular treatment is recommended for patients with documented lateralizing symptoms. Surgical correction is rarely indicated.
Dissection of the carotid artery accounts for approximately 20% of strokes in patients younger than 45 years of age. The etiology and pathogenesis of spontaneous carotid artery dissection remain incompletely understood. Arterial dissection involves hemorrhage within the media, which can extend into the subadventitial and subintimal layers. When the dissection extends into the subadventitial space, there is an increased risk of aneurysm formation. Subintimal dissections can lead to intramural clot or thrombosis. Traumatic dissection is typically a result of hyperextension of the neck during blunt trauma, neck manipulation, strangulation, or penetrating injuries to the neck. Even in supposedly spontaneous cases, a history of preceding unrecognized minor neck trauma is not uncommon. Connective disorders, such as Ehlers-Danlos syndrome, Marfan’s syndrome, α1-antitrypsin deficiency, or FMD, may predispose to carotid artery dissection. Iatrogenic dissections can also occur due to catheter manipulation or balloon angioplasty.
Typical clinical features of carotid artery dissection include unilateral neck pain, headache, and ipsilateral Horner’s syndrome in up to 50% of patients, followed by manifestations of the cerebral or ocular ischemia and cranial nerve palsies. Neurologic deficits can result either because of hemodynamic failure (caused by luminal stenosis) or by an artery-to-artery thromboembolism. The ischemia may cause TIAs or infarctions, or both. Catheter angiography has been the method of choice to diagnose arterial dissections, but with the advent of duplex ultrasonography, MRI/MRA, and CTA, most dissections can now be diagnosed using noninvasive imaging modalities (Fig. 23-25). The dissection typically starts in the internal carotid artery distal to the bulb. Uncommonly, the dissection can start in the common carotid artery or is an extension of a more proximal aortic dissection. Medical therapy has been the accepted primary treatment of symptomatic of carotid artery dissection. Anticoagulation (heparin and warfarin) and antiplatelet therapy have been commonly used, although there have not been any randomized studies to evaluate their effectiveness. The prognosis depends on the severity of neurologic deficit but is generally good in extracranial dissections. The recurrence rate is low. Therapeutic interventions have been reserved for recurrent TIAs or strokes or failure of medical treatment. Endovascular options include intra-arterial stenting, coiling of associated pseudoaneurysms, or, more recently, deployment of covered stents.
Carotid artery aneurysms are rare, encountered in less than 1% of all carotid operations (Fig. 23-26). The true carotid artery aneurysm is generally due to atherosclerosis or medial degeneration. The carotid bulb is involved in most carotid aneurysms, and bilaterality is present in 12% of the patients. Patients typically present with a pulsatile neck mass. The available data suggest that, untreated, these aneurysms lead to neurologic symptoms from embolization. Thrombosis and rupture of the carotid aneurysm are rare. Pseudoaneurysms of the carotid artery can result from injury or infection. Mycotic aneurysms often involved syphilis in the past, but are now more commonly associated with peritonsillar abscesses caused by Staphylococcus aureus infection. FMD and spontaneous dissection of the carotid artery can lead to the formation of true aneurysms or pseudoaneurysms. Whereas conventional surgery has been the primary mode of treatment in the past, carotid aneurysms are currently being treated more commonly using endovascular approaches.45
The carotid body originates from the third branchial arch and from neuro-ectodermal derived neural crest lineage. The normal carotid body is located in the adventitia or periadventitial tissue at the bifurcation of the common carotid artery (Fig. 23-27). The gland is innervated by the glossopharyngeal nerve. Its blood supply is derived predominantly from the external carotid artery but can also come from the vertebral artery. Carotid body tumor is a rare lesion of the neuroendocrine system. Other glands of neural crest origin are seen in the neck, parapharyngeal spaces, mediastinum, retroperitoneum, and adrenal medulla. Tumors involving these structures have been referred to as paraganglioma, glomus tumor, or chemodectoma. Approximately 5% to 7% of carotid body tumors are malignant. Although chronic hypoxemia has been invoked as a stimulus for hyperplasia of carotid body, approximately 35% of carotid body tumors are hereditary. The risk of malignancy is greatest in young patients with familial tumors.
Symptoms related to the endocrine products of the carotid body tumor are rare. Patients usually present between the fifth and seventh decades of life with an asymptomatic lateral neck mass. The diagnosis of carotid body tumor requires confirmation on imaging studies. Carotid duplex scan can localize the tumor to the carotid bifurcation, but CT or MRI is usually required to further delineate the relationship of the tumor to the adjacent structures. Classically, a carotid body tumor will widen the carotid bifurcation. The Shamblin classification describes the tumor extent: I, tumor is less than 5 cm and relatively free of vessel involvement; II, tumor is intimately involved but does not encase the vessel wall; and III, tumor is intramural and encases the carotid vessels and adjacent nerves.46 With good-resolution CT and MRI, arteriography is usually not required. However, arteriography can provide an assessment of the vessel invasion and intracranial circulation and allows for preoperative embolization of the feeder vessels, which has been reported to reduce intraoperative blood loss. Surgical resection is the recommended treatment for suspected carotid body tumor.
Blunt or penetrating trauma to the neck can cause injury to the carotid artery. Notwithstanding the massive bleeding from carotid artery transection, injury to the carotid artery can result in carotid dissection, thrombosis, or pseudoaneurysm formation. Carotid duplex ultrasound can be useful to locate the site of injury in the cervical segment of the carotid artery. Spiral CTA has become the modality of choice to detect extracranial carotid artery injury. Confirmation of carotid injury by contrast cerebral angiography remains the gold standard diagnostic test. Injuries to the cervical segment of the common and internal carotid arteries can be repaired surgically. Acute carotid artery thrombosis is usually treated medically with anticoagulation if the patient is asymptomatic. Revascularization should be considered for patients presenting with ongoing cerebral ischemia related to carotid artery thrombosis. Traumatic carotid artery dissection can cause cerebral ischemia due to thromboembolization, decreased flow, or thrombosis. Commonly, the dissection involves the distal portion of the cervical and petrous segment of the internal carotid artery. Medical management with antiplatelet or anticoagulation therapy is usually adequate for uncomplicated traumatic carotid dissection. In patients with pseudoaneurysms of the carotid artery that are located in a segment that is out of surgical reach, the use of selective coil embolization of the pseudoaneurysm or exclusion of the pseudoaneurysm by a covered stent graft has been reported. Bare metal stent has been used with success in the treatment of traumatic carotid artery dissection.
ABDOMINAL AORTIC ANEURYSM
Despite more than 50,000 patients undergoing elective repair of abdominal aortic aneurysm (AAA) each year in the United States, approximately 15,000 patients die annually as a result of ruptured aneurysm, making it the 10th leading cause of death in men in this country.47 The incidence appears to be increasing, and this is due in part to improvements in diagnostic imaging and, more importantly, a growing elderly population. With early diagnosis and timely intervention, aneurysm rupture–related death is largely preventable. Conventional treatment of an AAA involves replacing the aneurysmal segment of the aorta with a prosthetic graft, with the operation performed through a large abdominal incision. Techniques for this open abdominal surgery have been refined, adapted, and extensively studied by vascular surgeons over the past four decades. Despite a well-documented low perioperative mortality rate of 2% to 3% in large academic institutions, the thought of undergoing an open abdominal aortic operation often provokes a sense of anxiety in many patients due in part to the postoperative pain associated with the large abdominal incision as well as the long recovery time needed before the patient can return to normal physical activity.
The most common location of aortic aneurysms is the infrarenal aorta. Endovascular stent graft placement represents a revolutionary and minimally invasive treatment for infrarenal AAAs that only requires 1 to 2 days of hospitalization, and the patient can return to normal physical activity within 1 week. The concept of using an endoluminal device in the management of vascular disease was first proposed by Dotter and colleagues, who successfully treated a patient with iliac occlusion using transluminal angioplasty in 1964.48 Nearly two decades later, Parodi and colleagues reported the first successful endovascular repair of AAA using a stent graft device.15 Since then, a variety of stent graft technologies have been developed to treat AAA. The rapid innovation of this new treatment modality has undoubtedly captured the attention of patients with aortic aneurysms as well as physicians who practice endovascular therapy. Physicians in general should be knowledgeable regarding available treatment options of AAA in order to provide adequate evaluation and education to patients and their families. The purpose of this section is to outline the treatment options for AAAs, including conventional repair and endovascular approach. Advantages and potential complications of these treatments will also be addressed.
The pathogenesis of aneurysmal disease of the aorta is complex and multifactorial. A degenerative process in the aortic wall is the most common cause of AAA development.49 Matrix metalloproteinases (MMP), proteolytic enzymes, are found abundantly in the wall of AAA. Atherosclerotic disease, age, male sex, smoking history, family history, hypertension, coronary artery disease, and chronic obstructive pulmonary disease are associated with the development of AAA.50,51 Diabetes and black race have negative association with AAA.50 Other less common causes include inflammation, infection, and connective tissue disease. Inflammatory AAA accounts for 5% to 10% of all AAAs.52 In contrast to atherosclerotic AAA, the inflammatory variant is characterized pathologically by marked thickening of the aneurysm wall, fibrosis of the adjacent retroperitoneum, and rigid adherence of the adjacent structures to the anterior aneurysm wall.53 Male sex and smoking are even stronger risk factors in inflammatory AAA.54 Smoking cessation is the first step of medical therapy, followed by surgical repair. Infectious or mycotic AAA is rare but is associated with high mortality.55 Patients with connective tissue disorders such as Marfan’s syndrome and Ehlers-Danlos syndrome tend to have more extensive and larger aneurysms at a younger age.56
The natural history of an AAA is to expand and rupture. AAA exhibits a “staccato” pattern of growth, where periods of relative quiescence may alternate with expansion. Therefore, although an individual pattern of growth cannot be predicted, average aggregate growth is approximately 3 to 4 mm/year. There is some evidence to suggest that larger aneurysms may expand faster than smaller aneurysms, but there is significant overlap between the ranges of growth rates at each strata of size.
Rupture risk appears to be directly related to aneurysm size as predicted by Laplace’s Law. Although more sophisticated methods of assessing rupture risk based on finite element analysis of wall stress are under active investigation, maximum transverse diameter remains the standard method of risk assessment for aneurysm rupture. In the past, AAA rupture risk has been overestimated. More recently, two landmark studies have served to better define the natural history of AAA.57,58 Based on best available evidence, the annualized risk of rupture is given in Table 23-8. The rupture risk is quite low below 5.5 cm and begins to rise exponentially thereafter. This size can serve as an appropriate threshold for recommending elective repair provided one’s surgical mortality is below 5%. For each size strata, however, women appear to be at higher risk for rupture than men, and a lower threshold of 4.5 to 5.0 cm may be reasonable in good-risk patients. Although data are less compelling, a pattern of rapid expansion of >0.5 cm within 6 months can be considered a relative indication for elective repair. Aneurysms that fall below these indications may safely be followed with CT or ultrasound at 6-month intervals, with long-term outcomes equivalent to earlier surgical repair. Interestingly, in the Aneurysm Detection and Management (ADAM) study, 80% of all AAAs that were followed in this manner eventually came to repair within 5 years.58
DESCRIPTION | DIAMETER OF AORTA (CM) | ESTIMATED ANNUAL RISK OF RUPTURE (%) | ESTIMATED 5-YEAR RISK OF RUPTURE (%)a |
---|---|---|---|
Normal aorta | 2–3 | 0 | 0 (unless AAA develops) |
Small AAA | 4–5 | 1 | 5–10 |
Moderate AAA | 5–6 | 2–5 | 30–40 |
Large AAA | 6–7 | 3–10 | >50 |
Very large AAA | >7 | >10 | Approaching 100 |
Unless symptomatic or ruptured, AAA repair is a prophylactic repair. The rationale for recommending repair is predicated on the assumption that the risk of aneurysm rupture exceeds the combined risk of death from all other causes such as cardiopulmonary disease and cancer. On the other hand, our limitation in predicting timing and cause of death is underscored by the observation that over 25% of patients who were deemed unfit for surgical repair because of their comorbidities died from rupture of their aneurysms within 5 years.
Most AAAs are asymptomatic and are usually found incidentally during workup for chronic back pain or kidney stones. Physical examination is neither sensitive nor specific except in thin patients. Large aneurysms may be missed in the obese, while normal aortic pulsations may be mistaken for an aneurysm in thin individuals. Rarely patients present with back pain and/or abdominal pain with a tender pulsatile mass. Patients with these symptoms must be treated as a rupture until proven otherwise. If the patient is hemodynamically stable and the aneurysm is intact on a CT scan, the patient is admitted for blood pressure control with intravenous antihypertensive agents and undergoes repair usually within 12 to 24 hours or at least during the same hospitalization. In contrast, patients who are hemodynamically unstable with a history of acute back pain and/or syncope and a known unrepaired AAA or a pulsatile abdominal mass should be immediately taken to the operating room with a presumed diagnosis of a ruptured AAA.
Overall mortality of AAA rupture is 71% to 77%, which includes all out-of-hospital and in-hospital deaths, as compared with 2% to 6% for elective open surgical repair.59 Nearly half of all patients with ruptured AAA will die before reaching the hospital. For the remainder, surgical mortality is 45% to 50% and has not substantially changed in the last 30 years.
An AAA is defined as a pathologic focal dilation of the aorta that is greater than 30 mm or 1.5 times the adjacent diameter of the normal aorta (Fig. 23-28). Male aortas tend to be larger than female aortas, and there is generalized growth of the aortic diameter with each decade of life. Ninety percent of AAAs are infrarenal in location and have a fusiform morphology. There is a higher predilection for juxtarenal and suprarenal AAAs in women compared with men. Concomitant common iliac and/or hypogastric artery aneurysms can be found in 20% to 25% of patients. Although the etiology of most aortic aneurysms is atherosclerotic, clinically significant peripheral occlusive disease is unusual and present in less than 10% of all cases.
Although extravascular anatomy is important for open surgical repair of AAA, intravascular anatomy and aortoiliac morphology are important for endovascular repair. Pertinent anatomic dimensions include the diameter of the proximal nondilated infrarenal aortic neck, which can range from 18 to 30 mm; common iliac artery, which can range from 8 to 16 mm; and external iliac arteries, which can range from 6 to 10 mm. Morphologically, the aortic neck can manifest complex angulation above and below the renal arteries due to combination of elongation and anterolateral displacement by the posterior bulge of the aneurysmal aorta. Furthermore, the shape of the proximal neck is rarely tubular, but often is conical, reverse conical, or barrel-shaped. Distally, the iliac arteries can have severe tortuosity with multiple compound turns. Although significant from hemodynamic standpoint, severe iliac calcifications combined with extreme tortuosity can pose a formidable challenge during endovascular repair.
Preoperative evaluation should include routine history and physical exam with particular attention to (a) any symptoms referable to the aneurysm, which may impact the timing of repair; (b) history of pelvic surgery or radiation, in the event retroperitoneal exposure is required or interruption of hypogastric circulation is planned; (c) claudication suggestive of significant iliac occlusive disease; (d) lower extremity bypass or other femoral reconstructive procedures; and (e) chronic renal insufficiency or contrast allergy.
Cross-sectional imaging is required for definitive evaluation of AAA. Although ultrasound is safe, widely available, relatively accurate, and inexpensive and thus the screening modality of choice, CT scan remains the gold standard for determination of anatomic eligibility for endovascular repair. Size of AAA may differ up to 1 cm between CT and ultrasound, and during longitudinal follow-up, comparisons should be made between identical modalities. With modern multirow detector scanners, a timed-bolus intravenous contrast-enhanced, 2.5- to 3.0-mm slice spiral CT of the chest, abdomen, and pelvis can be performed in less than 30 seconds with a single breath hold. Extremely high-resolution images are obtained with submillimeter spatial resolution (Fig. 23-29). Proper window level and width (brightness and contrast) are important for discrimination among aortic wall, calcific plaque, thrombus, and lumen. The only major drawback to CT is the risk of contrast nephropathy in diabetics and in patients with renal insufficiency.
The spiral technique further affords the ability for three-dimensional reconstruction. Three-dimensional reconstructions can yield important morphologic information that is critical to endovascular therapy. Using third-party software, these images can be viewed and manipulated on one’s desktop computer, and so-called “center-line” (transverse slices perpendicular to the central flow lumen of the aorta) diameter and length measurements obtained. Conventional angiography has a minimal role in the current management of AAA. Angiography is invasive with an increased risk of complications. Indications for angiography are isolated to concomitant iliac occlusive disease (present in <10% of patients with AAA) and unusual renovascular anatomy.
General anesthesia is necessary when performing a conventional open AAA repair. While a retroperitoneal incision is a well-accepted surgical approach, a midline transabdominal incision remains the more common approach for open aortic aneurysm operation. Since the abdominal incision can lead to significant pain and discomfort, an epidural catheter can be placed prior to the operation for postoperative analgesic infusion to provide pain control. Once the abdominal cavity is opened, the small intestines and transverse colon are retracted to expose the retroperitoneum overlying the AAA. The retroperitoneum is next divided, followed by isolation of both proximal and distal segments of the AAA. Intravenous heparin (100 IU/kg) is given followed by clamping of the proximal and distal segments of the aneurysm. The aneurysm sac is open next, and a prosthetic graft is used to reconstruct the aorta. If the aneurysm only involves the abdominal aorta, a tube graft can be used to replace the aorta (Fig. 23-30). If the aneurysm extends distally to the iliac arteries, a prosthetic bifurcated graft is used for either an aorto-bi-iliac or aorto-bi-femoral bypass reconstruction (Fig. 23-31). The overlying aneurysm sac and the retroperitoneum are closed to cover the prosthetic bypass graft to minimize potential bowel contact to the graft. Small and large intestines are returned to the abdominal cavity followed by the closure of the abdominal fascia and skin.
The main advantage of a conventional open repair is that the AAA is permanently eliminated because it is entirely replaced by a prosthetic aortic graft. The risk of aneurysm recurrence or delayed rupture no longer exists. As a result, long-term imaging surveillance is not needed with these patients. In contrast, the long-term efficacy of endovascular repair remains unclear. Consequently, long-term imaging surveillance is critical to ensure that the aortic aneurysm remains properly sealed by the stent graft. Other potential advantages of open repair include direct assessment of the circulatory integrity of the colon. If signs of colonic ischemia become evident after aortic bypass grafting, a concomitant mesenteric artery bypass can be performed to revascularize the colonic circulation. In addition, open repair permits the surgeons to explore for other abdominal pathologies, such as gastrointestinal tumors, liver mass, or cholelithiasis.
As for the risks associated with open repair, cardiac complications, in the form of either myocardial infarction or arrhythmias, remain the most common morbidity, with an incidence between 2% and 6%.60 Another significant complication is renal failure or transient renal insufficiency as a result of perioperative hypotension, atheromatous embolization, inadvertent injury to the ureter, preoperative contrast-induced nephropathy, or suprarenal aortic clamping. Although the incidence of renal failure is less than 2% in elective aneurysm repair, it can occur in more than 20% of patients after repair of a ruptured AAA.60
Ischemic colitis is a devastating potential complication after open repair. The likelihood of such a complication is highest in those who had a prior colon resection and undergo repair of a ruptured AAA, due to the loss of collateral blood supply to the rectosigmoid colon. It is estimated that 5% of patients who undergo elective aneurysm repair will develop partial-thickness ischemic colitis but without significant clinical sequelae.61 However, if the partial-thickness ischemia progresses to full-thickness gangrene and peritonitis, mortality can be as high as 90%.61
The incidence of prosthetic graft infection ranges between 1% and 4% after open repair.61 It is more common in those who undergo repair of a ruptured AAA. If the prosthetic graft is not fully covered by the aneurysm sac or retroperitoneum, intestinal adhesion with subsequent bowel erosion may occur, resulting in an aortoenteric fistula. The predominant sign of such a complication is massive hematemesis, and it typically occurs years after the operation. Despite these potential complications, however, the majority of patients who undergo successful elective open repair have an uneventful recovery.
Over a decade has passed since the first report of human implantation of a homemade stent graft for endovascular repair of an AAA by Parodi in 1991.3 Several prospective clinical trials across different devices and analysis of large Medicare administrative databases and meta-analyses of published literature have consistently demonstrated significantly decreased operative time, blood loss, hospital length of stay, and overall perioperative morbidity and mortality of endovascular repair compared with open surgical repair. For patients who are at increased risk for surgery because of age or comorbidity, endovascular repair is a superior minimally invasive alternative.
The principle of endovascular repair of AAA involves the implantation of an aortic stent graft that is fixed proximally and distally to nonaneurysmal aortoiliac segment and thereby endoluminally excluding the aneurysm from the aortic circulation (Fig. 23-32). Unlike open surgical repair, the aneurysm sac is not resected, which is subjected for potential aneurysm expansion or even rupture. Importantly, aortic branches, such as lumbar arteries or the inferior mesenteric artery (IMA), are occluded, which can lead to persistent aneurysm pressurization and aneurysm expansion. Currently, the following nine devices are available for elective repair of intact infrarenal AAA: AneuRx device (Medtronic/AVE, Santa Rosa, CA), Gore Excluder device (WL Gore & Associates, Flagstaff, AZ), Endologix Powerlink device (Endologix Inc., Irvine, CA), Zenith device (Cook Inc., Bloomington, IN), Talent device (Medtronic/AVE, Santa Rosa, CA), Endurant device (Medtronic/AVE, Santa Rosa, CA), AFX Endovascular AAA System (Endologix Inc., Irvine, CA), Aorfix Flexible Stent (Lombard Medical Inc., Framingham, MA), and Ovation Prime Stent (TriVascular Inc., Santa Rosa, CA). Despite some differences in physical appearance, mechanical properties, and materials, they will be discussed collectively for this chapter. Most of these devices are modular devices consisting of a primary device or main body and one or two iliac limbs that insert into the main body to complete the repair. Depending on the device, there are varying degrees of flexibility in the choice of iliac limbs that can be matched to the main body, which can impact the customizability for a particular anatomy.
A severe limitation of the endovascular repair devices is the need for adequate proximal neck to achieve a durable sealing zone. Several techniques have been proposed to overcome this limitation. These include fenestrated or branched endografts and the “chimney,” “snorkel,” and “periscope” techniques. The fenestrated stent grafts rely on precise alignment between the fenestration and the corresponding visceral artery.62 Multiple clinical trials using customized fenestrated stent graft for the treatment of short-necked and juxtarenal aortic aneurysm repair have shown promising short- and mid-term results.63,64 However, restricted access to these investigational devices and the delays due to device customization limit the current use of these devices to a few centers. Alternatively, some centers have reported good results with intraoperative surgeon-modified endograft to create fenestrations for the treatment of complex aortic aneurysms in high-risk patients.65 Further development of the fenestrated techniques also opens the way for endovascular treatment of suprarenal and thoracoabdominal aneurysm.66 Recently, an “off-the-shelf” Zenith Fenestrated AAA endovascular graft has been approved by the FDA. This device has fenestrations or scallops in the graft material to allow the proximal edge of the stent graft to be placed above the renal arteries while still permitting blood flow to vessels accommodated by the fenestrations or scallops. The “chimney” technique involves placing renal or mesenteric stents parallel to the aortic stent graft to preserve blood flow in the visceral aortic branches.67 This technique has been suggested as a rescue procedure for visceral arteries that have been covered by the stent graft during endovascular repair.68 The review of literature showed that open surgery remains a safe and effective treatment option for good-risk patients with juxtarenal aortic aneurysm.69 Fenestrated endovascular repair is associated with low mortality and compares favorably with open surgery in terms of morbidity, especially renal function impairment and cardiac complications.69 The “chimney” technique demonstrated feasibility, but because of the limited number of reports and the lack of long-term data, it should be considered only in acute poor surgical risk patients and in case of unintentional visceral artery coverage or elective poor surgical cases for fenestrated endovascular repair.69
Anatomic eligibility for endovascular repair is mainly based on three areas: the proximal aortic neck, common iliac arteries, and external iliac and common femoral arteries, which relate to the proximal and distal landing zones or fixation sites and the access vessels, respectively. The requirements for the proximal aortic neck are a diameter of 18 to 28 mm and a minimum length of 15 mm (Table 23-9). Usually, multiple measurements of the diameter are taken along the length of the neck to assess its shape. All diameter measurements are mid-wall to mid-wall of the vessel. Secondary considerations include mural calcifications (<50% circumference), luminal thrombus (<50% circumference), and angulation (<45°). Presence of a significant amount of any one of these secondary features in combination with a relatively short proximal neck may compromise successful short- and long-term fixation of the stent graft and exclusion of the aneurysm. The usual distal landing zone is the common iliac artery. The external iliac artery may serve as an alternate site when the ipsilateral common iliac artery is aneurysmal or ectatic. The treatable diameters of common iliac arteries range from 8 to 20 mm, and there should be at least 20 mm of patent artery of uniform diameter to allow adequate fixation. Finally, at least one of two common femoral and external iliac arteries must be at least 7 mm in diameter in order to safely introduce the main delivery sheath. Slightly smaller iliac diameters may be tolerated depending on the specific device and in the absence of severe tortuosity and calcific disease. Difficult access is one of the main causes of increased procedural time and intraoperative complications. Using these criteria, approximately 60% of all AAAs are anatomic candidates for endovascular repair.
Neck length (mm) | >15 |
Neck diameter (mm) | >18, <32 |
Aortic Neck angle (degrees) | <60 |
Neck mural calcification (% circumference) | <50 |
Neck luminal thrombus (% circumference) | <50 |
Common iliac artery diameter (mm) | Between 8 and 20 |
Common iliac artery length (mm) | >20 |
External iliac artery diameter (mm) | >7 |
The next step in the preoperative planning is device selection. Typically, the proximal diameter of the main device is oversized by 10% to 20% of the nominal diameter of the aortic neck. Distally, the iliac limbs are oversized by 1 to 4 mm depending on the individual device’s instructions for use. The biggest challenge to proper device selection remains determining the optimal length from the renal arteries to the hypogastric arteries. Despite availability of sophisticated three-dimensional reconstructions, the exact path that a device will take from the proximal aortic neck to the distal iliac arteries is difficult to predict. It is dependent on a host of factors related to the mechanical properties of the stent graft and the morphology of the aortoiliac flow lumen. “Plumb-line” measurements of axial CT images can be quite inaccurate, typically grossly underestimating the length, whereas center-line measurements usually overestimate the length. Angiographic measurements using a marker catheter are invasive, require contrast and radiation exposure, and are also inaccurate because they fail to account for the stiffness of the stent graft. The consequences of not choosing the correct length of the device include inadvertent coverage of the hypogastric artery if too long and the need for additional devices if too short.
The obvious advantage of an endovascular AAA repair is its minimally invasive nature. Typically, patients who undergo this procedure stay in the hospital for only 1 to 3 days, in contrast to the 5- to 10-day stay required after conventional open surgical repair. In our institution, patients who have had an endovascular repair are routinely transferred to a general vascular ward from the postanesthesia recovery unit, avoiding admission to a more costly intensive care unit.
Because an abdominal incision is not necessary in endovascular repair, the procedure is particularly beneficial in patients with severe pulmonary disease, such as chronic obstructive pulmonary disease or emphysema. Patients can sustain adequate breathing in the postoperative period, avoiding respiratory complications or prolonged mechanical ventilation. Because the abdominal cavity has not been entered, the risk of gastrointestinal complications, such as ileus, ventral hernia, or bowel obstruction due to intestinal adhesion, is also greatly reduced. Moreover, regional or epidural anesthesia can be used, avoiding the risks associated with general anesthesia in patients with severe cardiopulmonary dysfunction.
Despite its many advantages, endovascular repair does have potential complications. Since the stent graft device is attached endoluminally within the abdominal aorta, an endoleak due to incomplete stent graft exclusion of the aneurysm can occur. With this type of leak, blood flow persists outside the lumen of the endoluminal graft but within an aneurysm sac. A meta-analysis of 1118 patients who underwent successful endovascular repair found an endoleak incidence of 24%.70 Although a small endoleak usually poses little clinical significance because it will typically become thrombosed spontaneously, a large or persistent endoleak may lead to continuous aneurysm perfusion and ultimately to aneurysm rupture. The rupture rate following an endovascular AAA repair has been reported to be less than 0.8%.71
Stent graft iliac limb dysfunction resulting in thrombosis has been reported following endovascular repair.18,70 One possible cause is aneurysm remodeling, resulting in a shortening in the aortic length, which can cause the stent graft to kink. Alternatively, progression of an underlying iliac atherosclerotic lesion may cause compression of the iliac limb and ultimately result in graft-limb occlusion. Treatment options include thrombolysis or graft thrombectomy to determine the underlying cause and possibly additional stent graft placement. Renal artery occlusion may occur due to improper stent graft positioning or migration.18,60,70 Graft limb separation or dislocation has also been reported.18,60,70
In patients with AAA and concurrent iliac artery aneurysms who undergo preoperative coil embolization of the internal iliac artery, 20% to 45% experience symptoms of pelvic ischemia.72 These symptoms may include buttock claudication, impotence, gluteal skin sloughing, and colonic ischemia. Other complications pertaining to endovascular repair relate to the access site and include groin hematoma and wound infection. Occasionally, the stent graft device can malfunction by either failing to deploy or dislodging during the deployment procedure.18,71 If the device cannot be salvaged or rescued endoluminally, open surgical repair of the aneurysm may be necessary.
Although endovascular AAA repair may be performed in any venue with appropriate digital fluoroscopic imaging capability, due to the need for absolute sterility and aseptic technique, it is most safely performed in a surgical suite. The patient is prepped and draped just as in open AAA repair. Patients with renal insufficiency should be started on perioperative oral N-acetylcysteine (Mucomyst) and sodium bicarbonate infusion to reduce the risk of contrast nephropathy. A variety of anesthetic options may be used. Regional anesthesia may be appropriate for patients with pulmonary disease. There are reports of success with local anesthetics alone, as the incisions are typically smaller than a typical open inguinal hernia repair.73
Bilateral transverse oblique incisions are made just below the inguinal ligament to expose approximately 2 to 3 cm of CFA and obtain proximal control. Special attention is paid to avoid the groin crease to decrease the risk of wound complications. Some have advocated a completely percutaneous access using the “pre-close” technique with the Perclose suture-mediated vascular closure device (Abbott Perclose, Redwood City, CA). Review of reported series on this technique suggest a technical success rate of 95% for medium-size sheaths ranging from 12 to 16 French, and 75% success for 18- to 24-French sizes.
Transfemoral access is obtained using standard Seldinger technique. Initial soft-tipped starter guidewires are exchanged for stiff guidewires that are advanced to the thoracic arch. Intravenous heparin at 80 IU/kg are administered, and the activated clotting time is maintained at 200 to 250 seconds. These guidewires provide the necessary support for the subsequent introduction of the large-diameter delivery catheters and devices. In the absence of special anatomic considerations, the primary device is inserted through the right side and the contralateral iliac limb is inserted through the left side. After administration of heparin, the delivery catheter or the introducer sheath is advanced to the L1-L2 vertebral space, which typically marks the location of the renal arteries. An angiographic catheter is advanced from the contralateral femoral artery to the same level.
A road-mapping aortogram is obtained to localize the renal arteries. The primary device is rotated to the desired orientation and deployed immediate below the lowest renal artery (Fig. 23-33). The angiographic catheter is replaced with a directional catheter and an angled guidewire, and the opening for the contralateral limb on the main device is cannulated. Intrastent passage of the guidewire is confirmed, and the angled guidewire is replaced with a stiff guidewire. The contralateral iliac limb is inserted into the docking opening of the primary device and deployed. A completion angiogram is performed looking for patency of the renal and hypogastric arteries, the device limbs, proximal and distal fixation, and endoleak. Adjunctive interventions including additional devices, balloons, and bare stents are performed as needed. The procedure is concluded with routine repairs of the femoral arteries and closure of the groin incisions. The patients recover in the recovery room for 2 to 4 hours and admitted to the general care floor. Although in the past, patients were admitted to the intensive care unit, this is rarely needed. Most patients can be started on a regular diet that evening and discharged the next morning.
Figure 23-33.
A. During an endovascular aortic aneurysm repair, the main endograft device is inserted through a femoral artery approach. B. The device is deployed in the aorta just below the renal arteries. C. A contralateral iliac endograft device is inserted through a contralateral gate opening, which is next deployed. D. Completion of deployment of the endograft device should fully exclude an aortic aneurysm while preserving flow of the renal and hypogastric arteries.
Life-long follow-up is essential to the long-term success after endovascular AAA repair. Indeed, one may go so far as to say that absence of appropriate follow-up is tantamount to not having had a repair at all. A triple-phase (noncontrast, contrast, and delayed) spiral CT scan and a four-view (anteroposterior, lateral, and two obliques) abdominal x-ray should be obtained within the first month. Subsequent imaging can be obtained at 6-month intervals in the first 1 to 2 years and yearly thereafter. After the first 6 months, patients who cannot travel easily may obtain their studies locally and submit them for review. The CT scan is for detection of endoleaks, subtle proximal migrations, and changes in aneurysm size. The abdominal x-ray gives a “birds-eye” view of the overall morphology of the stent graft. Subtle changes in conformation of the iliac limbs relative to each other and/or the spine can provide early signs of impending component separation or loss of fixation. Further, stent fractures and/or suture breaks that can compromise long-term device integrity can sometimes only be detected on a plain film and not on a CT scan.
The primary success rate after endovascular repair of AAA has been reported to be as high as 95%.18,59 The less invasive nature of this procedure is appealing to many physicians and patients. In addition, virtually all reports indicate a decreased blood loss, transfusion requirements, and length of intensive care unit and hospital stay for endovascular repair of AAAs compared with the standard surgical approach.18,59,74 With the advent of bifurcated grafts and improved delivery systems in the future, the only real limitation will be cost. When evaluating the literature for results from clinical series, it is important to look at a comparison of endoluminal versus open repair and device-specific outcome and cost analysis studies.
Early reports on results with endovascular repair were often flawed due to selection biases. This is because from its inception, endovascular repair has been used mostly in patients who are at higher risk for open repair. At the same time, only patients with favorable anatomy including less tortuosity and the presence of a suitable infrarenal neck were considered for endovascular repair. Randomization is also difficult because most patients who anatomically qualify for endovascular repair would withdraw from the study if randomized to open repair. Consequently, there are very few randomized controlled trials that have compared outcomes in patients with similar risk factors and anatomy who are eligible for both types of repair. Two such European trials have recently published short-term outcome data that are unbiased in design.
The DREAM trial is a multicenter randomized trial that compared open versus endovascular repair among a group of 345 patients at 28 European centers using multiple different devices including Gore, AneuRx, and Zenith.75 Patients were included only if they were considered to be candidates for both types of repairs. The operative mortality rate was 4.6% in the operative group versus 1.2% in the endoluminal group at 30 days. When looking at the combined rate of operative mortality and severe complications, there was an incidence of 9.8% in the open repair group versus 4.7% in the endoluminal group. The difference here was largely due to the higher frequency of pulmonary complications seen in the open group. There was a higher incidence of graft-related complications in the endoluminal group. There was no difference in the nonvascular local complication rate among the two groups. The Endovascular Repair-1 (EVAR-1) trial is also a multicenter randomized trial that compared open to endoluminal repair.74 This study was conducted on 1082 patients at 34 centers in the United Kingdom using all available devices. Short-term mortality at 30 days was 4.7% in the open group and 1.7% in the endoluminal group. The in-hospital mortality rate was also increased in the open when compared to the endoluminal group (6.2% vs. 2.1%). As expected, the secondary intervention rate was higher in the endoluminal group (9.8% vs. 5.8%). Complication rates were not reported in the EVAR-1 trial. Criticisms can be applied to both of these trials. Patients had to be eligible for either type of repair in order to be included in the study. Consequently, these findings cannot be generalized to patients who are too sick to undergo open surgery or to patients whose anatomy precludes them from undergoing endovascular repair.
The Open Versus Endovascular Repair (OVER) Veterans Affairs Cooperative Study Group randomly assigned 881 patients with asymptomatic AAAs who were candidates for both procedures to either endovascular repair (n = 444) or open repair (n = 437) and followed them for up to 9 years.76 Reduction in perioperative mortality with endovascular repair was sustained at 3 years but not thereafter. There was no difference in primary outcome of all-cause mortality. Endovascular repair and open repair resulted in similar long-term survival. Six aneurysm ruptures were confirmed in the endovascular repair group versus none in the open repair group. Rupture after endovascular repair remains a concern. A significant interaction was observed between age and type of treatment. Endovascular repair led to increased long-term survival among younger patients but not among older patients, for whom a greater benefit from the endovascular approach had been expected.
Matsumura and associates compared endoluminal versus open repair using the Excluder device.77 In their review, they demonstrated a 30-day mortality rate of 1% along with endoleak rates of 17% and 20% at 1- and 2-year intervals, respectively.77 The limb narrowing, limb migration, and trunk migration were all 1% at 2 years. There were no deployment failures or early conversions. There was an annual 7% reintervention rate. Aneurysm growth was demonstrated in 14% of patients at 2 years. The Zenith device by Cook has been studied by Greenberg and associates, who compared standard surgical repair with endoluminal repair in low-risk patients and endoluminal repair in high-risk patients.78 They reported a 30-day mortality rate of 3.5%, which was equal to the open group. The endoleak rates were 7.4% and 5.4% at 1- and 2-year intervals, respectively. There was a 5.3% migration of 5 mm at 1 year. Freedom from rupture was 100% in the low-risk group and 98.9% in the high-risk endoluminal group at 2 years. Experience with the AneuRx device has been reported by Zarins.79 In this 4-year review, they found a 30-day mortality rate of 2.8%. Endoleak rate at 4 years was 13.9%, aneurysm enlargement was 11.5%, and stent graft migration was 9.5%. Freedom from rupture was noted to be 98.4% at 4 years. Criado and associates have reported on their 1- year experience with the Talent LPS device by Medtronic.80 They report a 30-day mortality rate of 0.8%. Endoleak rate was 10%. Three deployment failures were noted, and freedom from rupture was 100%. Aneurysm growth and migration rates were divided into three different neck size groups. Patients with a wide neck (>26 mm) had a 3% growth and migration rate. Narrow-neck patients (<26 mm) had a 1% growth rate and a 2% migration neck. Interestingly, short-neck patients (<15 mm) had no aneurysm growths and a 2% migration rate.
The current climate of cost containment and limited reimbursement for healthcare services mandates a critical analysis of the economic impact of any new medical technology on the market. The in-hospital costs for both endovascular and open repair include graft cost, operating room fees, radiology, pharmacy, ancillary care, intensive care unit charges, and floor charges. Despite the improved morbidity and mortality rates, several early studies have reported no cost benefit with the application of endovascular repair.81,82 The limiting factor appears to be the cost of the device. Despite commercialization of endovascular repair, the device costs are still in the range of $5000 to $6000 with no signs of abating. A recent report by Angle and associated further corroborates previous studies.83 In their review, despite decreased hospital and intensive care unit stays and utilization of pharmacy and respiratory services, cost of endovascular repair was 1.74 times greater than the standard surgical approach. In addition, these cost analysis studies are centered on in-hospital costs and do not even begin to address secondary costs such as postoperative surveillance that is required with endovascular repair. In the OVER trial, endovascular repair was found to be a cost-effective alternative to open repair in the U.S. Veterans Affairs healthcare system for at least the first 2 years.84 The primary outcomes were mean total healthcare cost per life-year and per quality-adjusted life-year. There were no differences found in survival, quality of life, and costs after 2 years between the endovascular and the open group. Although graft costs were higher in the endovascular group, length of stay was shorter, resulting in lower cost of AAA repair hospitalization in the endovascular group. Costs remained lower after 2 years in the endovascular group, but the difference was no longer significant.
An endoleak is an extravasation of contrast outside the stent graft and within the aneurysm sac (Fig. 23-34). It can be present in up to 20% to 30% of all endovascular AAA repairs in the early postoperative period.85 In general, over half of these endoleaks will resolve spontaneously during the first 6 months, resulting in a 10% incidence of chronic endoleaks in all cases beyond the first year of follow-up. Endoleaks can be detected using conventional angiography, contrast CT (Fig. 23-35), MRA, and color-flow duplex ultrasound. Although there is no recognized gold standard, in practice, angiography is considered the least sensitive but most specific for characterizing the source of the endoleak, whereas the CT scan is the most sensitive but least specific. Widespread availability and reliability that is relatively independent of technique have made the CT scan the de facto standard imaging modality for postoperative surveillance. Conversely, routine use of duplex ultrasound and MRA has been limited by the lack of proper equipment and local expertise. On the other hand, investigational techniques such as time-resolved MRA may provide greater sensitivity and specificity than either angiography or CT in the future.
Figure 23-34.
The four types of endoleak include the following: type I endoleak = attachment site leak; type II endoleak = side branch leak caused by lumbar or side branches; type III endoleak = endograft junctional leak due to overlapping device components; and type IV endoleak = endograft fabric or porosity leak.
Four types of endoleaks have been described (Table 23-10). Type I endoleak refers to fixation-related leaks that occur at the proximal or distal attachment sites. These represent less than 5% of all endoleaks and are seen as an early blush of contrast into the aneurysm sac from the proximal or distal ends of the device during completion angiography.85,86 Although seen as marker of poor patient selection or inadequate repair, over 80% of these leaks spontaneously seal in the first 6 months. Persistent type I endoleaks, on the other hand, require prompt treatment. Type II endoleak refers to retrograde flow originating from a lumbar, inferior mesenteric, accessory renal, or hypogastric artery. They are the most common type of endoleak, accounting for 20% to 30% of all cases, and about half resolve spontaneously. On angiography, they are seen as a late filling of the aneurysm sac from a branch vessel(s). Type II endoleaks carry a relatively benign natural history and do not merit intervention unless associated with aneurysm growth. Type III endoleaks refer to failure of device integrity or component separation from modular systems. If detected intraoperatively or in the early perioperative period, it is usually from inadequate overlap between two stent grafts, whereas in the late period, the endoleak may be from a fabric tear or junctional separation from conformational changes of the aneurysm. Regardless of the etiology or timing, these should be promptly repaired. Finally, type IV endoleak refers to the diffuse, early blush seen during completion angiography due to graft porosity and/or suture holes of some Dacron-based devices. It does not have any clinical significance and usually cannot be seen after 48 hours and heparin reversal. Endoleaks that are initially considered type IV but persist become type III endoleaks by definition, because this indicates a more significant material defect than simple porosity or a suture hole.
CLASSIFICATION | DESCRIPTION |
---|---|
Type I endoleak | Attachment site leak |
Type II endoleak | Side branch leak caused by lumbar or inferior mesenteric arteries |
Type III endoleak | Junctional leak (of overlapping endograft components) and graft fabric defect |
Type IV endoleak | Endograft fabric porosity leak |
In approximately 5% of cases after an apparently successful endovascular repair, the aneurysm continues to grow without any demonstrable endoleak.87 This phenomenon has been described as endotension. Although it was initially thought that an endoleak was really present but simply not detected, case have been reported where the aneurysm has been surgically opened and the contents were completely devoid of any blood and no extravasation could be found. The mechanism of continued pressurization of the aneurysm sac following successful exclusion from the arterial circulation remains unsolved at this time. One putative mechanism has been linked to a transudative process related to certain expanded PTFE graft materials.88 More importantly, however, the natural history of these enlarging aneurysms without endoleaks is unknown, but to date, there has been no evidence to suggest that they carry an increased risk of rupture. Conservatively speaking, until further long-term data become available, if the patient is a suitable surgical risk, elective open conversion should be considered.
There is approximately 10% to 15% per year risk of secondary interventions following endovascular AAA repair.18,78,89 These procedures are critical in the long-term success of the primary procedure in prevention of aneurysm rupture and aneurysm-related death. These secondary procedures, in order of frequency, include proximal or distal extender placement for migrations, highly selective or translumbar embolization for type II endoleaks, direct surgical or laparoscopic branch vessel ligations, bridging cuffs for component separations, and late open surgical conversions.
Multiple large series have reported that an annual rupture rate of approximately 1% to 1.5% per year after endovascular repair.18,78,89 The EUROSTAR registry reports a rupture rate of 2.3% over 15.4 months in patients with an endoleak, compared with 0.3% in those without.90 Various causes of late ruptures have been reported in the literature, although presence of a persistent endoleak with aneurysm enlargement remains a common culprit for this complication. It has been shown that even successfully excluded aneurysms can lead to the development of attachment-site leaks and device failure, caused in part by aneurysm remodeling resulting in stent migration or kinking.91 Mehta and colleagues reported that 63% of delayed AAA ruptures after endovascular repair were caused by type I endoleaks with endograft migration, 11% by type I without migration, 19% by type II, and the rest of unknown type.92
Treatment of rupture may be open conversion or endovascular stent graft placement. May and associates reported a mortality rate of 43% in those patients who underwent open conversion.93 Emergent endovascular repair should be considered in these patients since it is potentially much faster and less likely to cause physiologic stress than open conversion. Several reports have shown that endovascular repair can be performed successfully in patients previously treated with endoluminal prostheses.94,95
MESENTERIC ARTERY DISEASE
Vascular occlusive disease of the mesenteric vessels is a relatively uncommon but potentially devastating condition that generally presents in patients over 60 years of age, is three times more frequent in women, and has been recognized as an entity since 1936.96 The incidence of such a disease is low and represents 2% of the revascularization operations for atheromatous lesions. The most common cause of mesenteric ischemia is atherosclerotic vascular disease. Autopsy studies have demonstrated splanchnic atherosclerosis in 35% to 70% of cases.97 Other etiologies exist and include FMD, panarteritis nodosa, arteritis, and celiac artery compression from a median arcuate ligament, but they are unusual and have an incidence of one in nine compared with that of atherosclerosis.
Chronic mesenteric ischemia is related to a lack of blood supply in the splanchnic region and is caused by disease in one or more visceral arteries: the celiac trunk, the superior mesenteric artery, and the IMA. Mesenteric ischemia is thought to occur when two of the three visceral vessels are affected with severe stenosis or occlusion; however, in as many as 9% of cases, only a single vessel is involved (SMA in 5% and celiac trunk in 4% of cases).98 This disease process may evolve in a chronic fashion, as in the case of progressive luminal obliteration due to atherosclerosis. On the other hand, mesenteric ischemia can occur suddenly, as in the case of thromboembolism. Despite recent progress in perioperative management and better understanding of pathophysiology, mesenteric ischemia is considered one of the most catastrophic vascular disorders with mortality rates ranging from 50% to 75%. Delays in diagnosis and treatment are the main contributing factors in its high mortality. It is estimated that mesenteric ischemia accounts for 1 in every 1000 hospital admissions in this country. The prevalence is rising due in part to the increased awareness of this disease, the advanced age of the population, and the significant comorbidity of these elderly patients. Early recognition and prompt treatment before the onset of irreversible intestinal ischemia are essential to improve the outcome.
Mesenteric arterial circulation is remarkable for its rich collateral network. Three main mesenteric arteries provide the arterial perfusion to the gastrointestinal system: the celiac artery (CA), the superior mesenteric artery (SMA), and the IMA. In general, the CA provides arterial circulation to the foregut (distal esophagus to duodenum), hepatobiliary system, and spleen; the SMA supplies the midgut (jejunum to mid-colon); and the IMA supplies the hindgut (mid-colon to rectum). The CA and SMA arise from the ventral surface of the infradiaphragmatic suprarenal abdominal aorta, whereas the IMA originates from the left lateral portion of the infrarenal aorta. These anatomic origins in relation to the aorta are important when a mesenteric angiogram is performed to determine the luminal patency. In order to fully visualize the origins of the CA and SMA, it is necessary to perform both an anteroposterior and a lateral projection of the aorta since most arterial occlusive lesions occur in the proximal segments of these mesenteric trunks.
Because of the abundant collateral flow between these mesenteric arteries, progressive diminution of flow in one or even two of the main mesenteric trunks is usually tolerated, provided that uninvolved mesenteric branches can enlarge over time to provide sufficient compensatory collateral flow. In contrast, acute occlusion of a main mesenteric trunk may result in profound ischemia due to lack of sufficient collateral flow. Collateral networks between the CA and the SMA exist primarily through the superior and inferior pancreaticoduodenal arteries. The IMA may provide collateral arterial flow to the SMA through the marginal artery of Drummond, the arc of Riolan, and other unnamed retroperitoneal collateral vessels termed meandering mesenteric arteries (Fig. 23-36). Lastly, collateral visceral vessels may provide important arterial flow to the IMA and the hindgut through the hypogastric arteries and the hemorrhoidal arterial network.
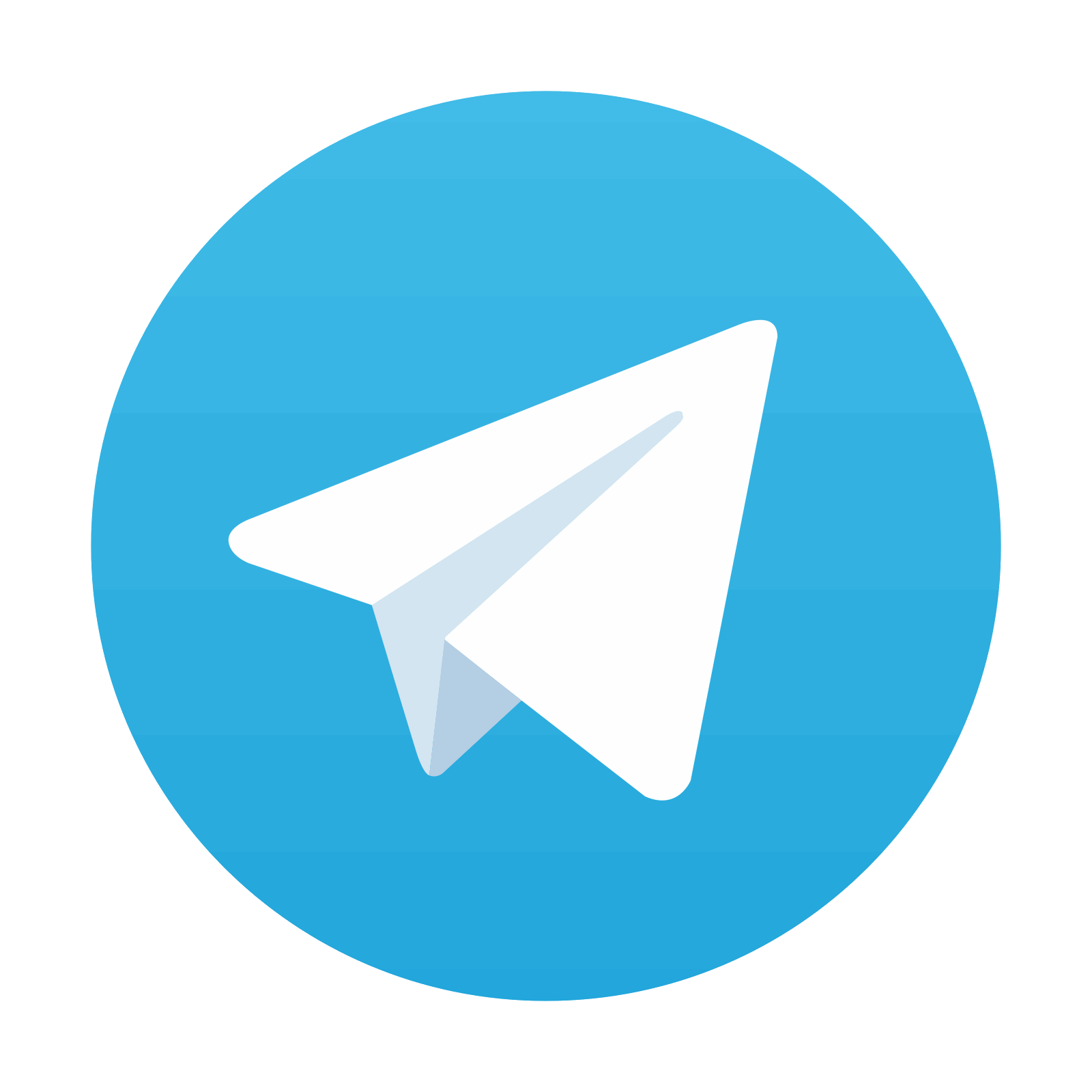
Stay updated, free articles. Join our Telegram channel

Full access? Get Clinical Tree
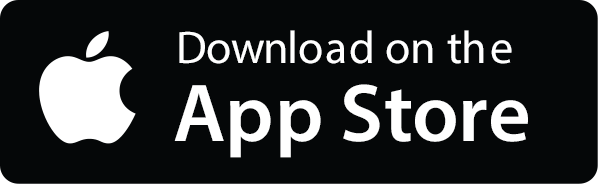
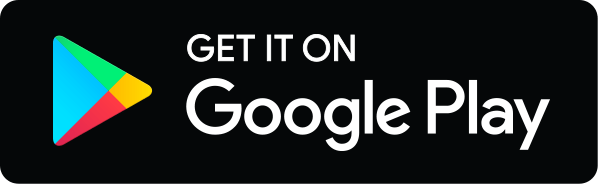