Antibodies in COVID-19
Mehak Zahoor Khan
Rachel Leeson
Carole Henry
Galit Alter
INTRODUCTION
Antibodies, or immunoglobulins (Igs), were first mentioned in the literature in 1890, when Emil von Behring and Shibasaburo Kitasato published diphtheria serum transfer studies, referring to neutralizing agents, or “antikörper,” in the blood of immunized animals that conferred pathogen-specific immunity.1,2 A decade later, Paul Ehrlich proposed the side-chain theory, describing antibodies as branched molecules containing multiple “lock-and-key” binding sites. Although it was incorrectly described as “chains” that grew out from the cell, the lock-and-key mechanism was confirmed in the 1940s by Linus Pauling,3 who demonstrated the exquisite specificity conferred by these serum proteins. Molecular structural work on antibodies was published in 1959, in independent studies from Gerald Edelman and Rodney Porter.4,5 In 1948, Astrid Fagraeus deepened the understanding of the source of these molecules, demonstrating the highly specialized role of plasma cells that produce antibodies. This was followed by the publication by Frank Burnet and David Talmage describing clonal selection theory in 1957,6 a theory that was experimentally confirmed in a 1958 publication by Gustav Nossal and Joshua Lederberg.7 However, the major explosion in antibody research occurred after Georges Köhler and César Milstein developed a method to produce monoclonal antibodies (mAbs) in vitro,8 in 1975, greatly expanding our ability to study antibodies and explore their therapeutic and research applications. mAbs now represent the fastest growing class of drugs,9,10 with
hundreds of therapeutics in clinical development and testing across disease indications from autoimmunity to infectious disease. The coronavirus disease 2019 (COVID-19) pandemic illustrated the critical role of antibodies in the prevention of disease, in the setting of prophylactic vaccination, and as therapeutics for the treatment of disease. Here, we will review the anatomy of antibodies, their origin, their role in vaccination, and the promise of next-generation strategies through the lens of severe acute respiratory syndrome coronavirus 2 (SARS-CoV-2).
hundreds of therapeutics in clinical development and testing across disease indications from autoimmunity to infectious disease. The coronavirus disease 2019 (COVID-19) pandemic illustrated the critical role of antibodies in the prevention of disease, in the setting of prophylactic vaccination, and as therapeutics for the treatment of disease. Here, we will review the anatomy of antibodies, their origin, their role in vaccination, and the promise of next-generation strategies through the lens of severe acute respiratory syndrome coronavirus 2 (SARS-CoV-2).
ANTIBODIES
Antibodies are Y- or T-shaped polypeptide proteins, comprised of four peptide chains: two heavy chains and two light chains (Figure 8.1). Together, these four chains form two identical antigen-binding fragments (Fabs) and a crystallizable fragment (Fc), linked by a hinge domain.11 They are expressed in B cells, acting as B-cell receptors (BCRs), and produced by antibody-secreting cells, including short-lived plasmablasts and long-lived plasma cells.12 Each B cell has a unique BCR, formed via a random recombination event during B-cell maturation in the bone marrow, resulting in the generation of up to 1012 unique BCRs with distinct antigen recognition capabilities.13 At the start of the maturation process, one of two light chains (κ or λ) is selected to form the antibody Fab. The light chains pair with the heavy chains to form a polypeptide of two light chains and two heavy chains, forming two identical antigen-binding sites. Variation in the antigen-binding sites arises from the recombination of VJ (light chain) and VDJ (heavy chain) segments found in the Ig locus, and the ensuant random repair following end-joining creates random variation that collectively forms the complementarity determining regions (CDRs),14 which are supported by framework regions found on the end of each of the polypeptide chains.14,15 The CDRs are responsible for antibody specificity and variation.
![]() FIGURE 8.1 The structure of an antibody. The inset shows the CDR loops and framework (FR) of the variable region. CDR, complementarity determining region. |
Typically, B cells encounter and sample antigens with their BCR in dedicated regions of the lymph node called germinal centers (GCs),16, 17 and 18 where antigens are arrayed on specialized set of fibroblasts known as follicular dendritic cells (fDCs).19 When the BCRs productively engage an antigen, B cells rapidly become activated, acquiring signals to proliferate, travel to a newly developed dark zone of the GC, and begin hypermutating their BCR.20 Hypermutation involves the specialized incorporation of additional mutations in the CDRs of the antibody, aimed at randomly allowing for the creation of almost unlimited variability in antigen specificity and affinities.14 Hypermutated daughter cells then circulate back to the light zone of the GC, resampling the arrayed antigen. Cells that bind the antigen more tightly gain survival and proliferative signals, present the processed antigen to follicular T-helper cells (Tfh), and return to the dark zone for further hypermutation. Cells that do not gain affinity, and therefore do not receive additional signals, die off. Following several mutational rounds, a subset of B cells differentiates into short-lived plasmablasts primed to populate the system with antibodies. A second subset of high-affinity GC B cells differentiate into plasma cells that take up long-term residence in the bone marrow. Finally, a third subset become memory B cells that circulate widely through the body, providing memory upon pathogen/antigen reencounter.
The Fc-domain, which is composed of two polypeptide chains, can also change during the life span of a B cell. In humans, the Ig locus contains nine different potential Fc-domains that can be attached to any Fab. These include the five antibody isotypes, IgD, IgM, IgG, IgA, and IgE, in which IgG and IgA are composed of the subclasses IgG1, IgG2, IgG3, and IgG4, and IgA1 and IgA2, respectively21,22 (Table 8.123). Each Fc-domain can bind to isotype-specific Fc-receptors (FcRs) found in different combinations and at different levels across cells of the immune system.24,25 Specific inflammatory cues and T-helper signals, present at the time of B-cell programming, lead to the selection of a particular Fc-domain able to recruit and drive the most appropriate immunologic functions to clear the pathogen the antibody recognizes, that is, mucosal pathogens tend to drive IgA Fc-selection, known to play a critical role at mucosal barriers, whereas systemic viral infections tend to elicit IgG1/IgG3 responses that have high affinity for FcRs present on systemic immune cells.22,24 Together, the pairing of the optimal Fab and Fc results in the generation of bifunctional molecules able to fully leverage the immune system to fight disease.
TABLE 8.1 Summary of major classes of antibodies in humans | ||||||||||||||||||||||||||||||||||||||||||||||||||||||||||||
---|---|---|---|---|---|---|---|---|---|---|---|---|---|---|---|---|---|---|---|---|---|---|---|---|---|---|---|---|---|---|---|---|---|---|---|---|---|---|---|---|---|---|---|---|---|---|---|---|---|---|---|---|---|---|---|---|---|---|---|---|
|
FC-RECEPTORS
The Fc-domain of an antibody plays two critical roles. First, it provides instructions to the immune system on how the antigen to which the antibody is bound should be destroyed. Second, the Fc-domain dictates the half-life of the antibody. Interaction of the antibody Fc-domain with a range of FcRs, found across immune and nonimmune cells, dictates these two functions. Individual FcRs, expressed on a variety of innate immune cell types, exist for nearly all antibody isotypes and subclasses, allowing each to deploy specific immunologic functions (Table 8.2). For example, the Fcμ-receptor (FcµR) has been implicated in opsonophagocytosis and T-cell activation.26,27 FcαR expression is more restricted, but it can activate a large number of functions. For example, FcαRI activation can result in phagocytosis, degranulation, superoxide generation, release of neutrophil extracellular traps (NETs), antibody-dependent cellular cytotoxicity (ADCC), release of cytokines and chemokines, or antigen presentation.28 A diverse set of activating and inhibitory FcγRs, expressed across distinct immune cell types, can deploy a range of immunologic functions that may be essential to the elimination of pathogens or aberrant cells/materials in the body. All FcRs except FcγRIIIB possess or are associated with signaling receptors that provide activating or inhibitory signals. FcγRIIIB is a Glycosylphosphatidylinositol (GPI)-anchored protein, which captures antibodies largely on neutrophils.
TABLE 8.2 Summary of IgG Fc-receptors in humans | ||||||||||||||||||||||||||||||||||||||||||||||||
---|---|---|---|---|---|---|---|---|---|---|---|---|---|---|---|---|---|---|---|---|---|---|---|---|---|---|---|---|---|---|---|---|---|---|---|---|---|---|---|---|---|---|---|---|---|---|---|---|
|
Although FcRs for IgE and IgA exhibit high affinity for their antibodies, FcγRI is a high-affinity receptor and the remaining four FcγRs—FcγRIIA, FcγRIIB, FcγRIIIA, and FcγRIIIB—are all low-affinity receptors,29 requiring multimerization to activate cells.30 This affinity threshold across the FcγRs acts as a quality control and ensures the appropriate activation threshold is met on innate immune cells prior to cellular activation, preventing pathologic activation of the innate immune system.
Antibody half-life is dictated by the ability of the antibody Fc-domain to interact with the neonatal FcR (FcRn).31,32 FcRn is expressed across both endothelial cells and a subset of immune cells. In nonimmune cells, antibody binding results in antibody capture and recycling back into the circulation.33 FcRn is also involved in transferring antibodies across barriers, such as the placental or blood-brain barrier, to ensure the presence of antibodies across tissues and compartments.34,35 IgG
antibodies bind to FcRn with a higher affinity and thus they both persist for longer periods of time and can be transferred more efficiently to tissues. In immune cells, FcRn is involved in driving antigen processing and presentation for the potent induction of T-cell immune responses. Finally, FcRn also contributes to the delivery of antibody-antigen/pathogen complexes to antigen-presenting cells (APCs) that are involved in T-cell education.36
antibodies bind to FcRn with a higher affinity and thus they both persist for longer periods of time and can be transferred more efficiently to tissues. In immune cells, FcRn is involved in driving antigen processing and presentation for the potent induction of T-cell immune responses. Finally, FcRn also contributes to the delivery of antibody-antigen/pathogen complexes to antigen-presenting cells (APCs) that are involved in T-cell education.36
ANTIBODY EFFECTOR FUNCTIONS
Antibodies act through synergy, typically binding a target as a polyclonal pool of molecules, meaning that antibodies with different affinities, geometries, and Fc-domains bind simultaneously to a target, forming a large complex, also known as an immune complex (IC). Thus, given the multitude of different Fc-domains that may be present within a given IC, antibodies can drive a range of different functional responses. In viral infection, these effector functions include either direct antiviral activity through neutralization or indirect antiviral activity through the recruitment of immune functions via Fc:FcR interactions.
Direct neutralization occurs when neutralizing antibodies (nAbs) bind to a pathogen and physically prevent the pathogen from infecting a new cell. nAbs often bind to the receptor or close to the receptor used by the pathogen to attach to the host cell, such as the receptor-binding domain (RBD) on SARS-CoV-2, preventing viral binding to the host cell, and thereby preventing entry and thus infection. As this is largely driven by the Fab-domain, all isotypes and subclasses of antibodies can be nAbs. However, viruses often evolve rapidly to evade direct nAbs. Thus, the Fab must perpetually evolve to catch-up to pathogen evolution. For example, over the COVID-19 pandemic, several variants emerged that were able to evade the nAbs induced by various vaccines.37 Although the vaccine-induced immune responses, programmed with the original SARS-CoV-2 Spike antigen, were insufficient to provide protection against the new variants, the ensuing humoral immune responses observed in breakthrough vaccine cases evolved to cover the new variants, illustrating the plasticity of the memory B-cell response able to rapidly adapt to an evolving pathogen (see Figure 8.2).
FC-MEDIATED EFFECTOR FUNCTIONS
ADCC is the antibody-driven killing of target cells by natural killer (NK) cells, lymphocytes that belong to the innate arm of the immune system, and represent the first line of defense against incoming pathogens. NK cells recognize their targets via an array of receptors that detect transformed or infected cells.38 Mature NK cells express copious amounts of FcRs, specifically the FcγRIIIA receptor, enabling a pathogen-specific response to antibody-opsonized targets. Upon activation by IgG/FcγRIIIA, NK cells can release cytotoxic granules containing perforin, which creates channels in the cell membrane, and granzyme, which drives apoptosis in the cell. Although NK cells are primarily responsible for ADCC in vivo, multiple other cytotoxic innate effector cells, including neutrophils, monocytes, macrophages, and NK T cells, have been shown to also induce ADCC in vitro. ADCC has been implicated in the control of influenza39,40 and human immunodeficiency virus (HIV),41, 42 and 43 and has also been observed in the setting of SARS-CoV-2 infection.44, 45 and 46
Antibody-dependent cellular phagocytosis (ADCP), or opsonophagocytosis, is the antibody-mediated mechanism whereby antibodies drive pathogen-antibody complex uptake into phagocytic cells, where the complexes are degraded in the lysosomes. Phagocytosis can be induced by a wide range of receptors, including high- and low-affinity receptors, as well as by activating and inhibitory receptors.47 Monocytes, macrophages, neutrophils, B cells, and dendritic cells (DCs) can all mediate opsonophagocytic uptake of ICs. Depending on the ligated cell type, ADCP can induce cytokine release, neutrophil NETosis (a process in which neutrophils release extracellular webs of chromatin, antimicrobicidal proteins, and oxidant enzymes that kill extracellular pathogens48,49), antigen presentation by DCs to promote more effective T-cell immunity, and/or antiviral mechanisms. ADCP may play a role in the immune response to HIV,50,51 adenovirus,52 West Nile virus,53 and coxsackieviruses.54,55
Antibody-mediated complement deposition, also known as complement-dependent cytotoxicity (CDC), occurs when antibodies activate the classical complement pathway, enabling the
first member of the cascade, C1q, to deposit on the surface of the pathogen.56 C1q, a pentamer of C1 molecules, can interact with IgM (also a pentamer) or multimerized IgG3 and IgG1 antibodies via their Fc-domains, once they have bound to the surface of a pathogen.57,58 C1q binding then leads to a cascade of enzymatic reactions and catalytic events, wherein additional members of the complement cascade deposit, forming the membrane attack complex (MAC) that ruptures the membrane of the pathogen and death. The deposition of complement components, midway in the formation of the MAC, such as C3b or C5b, can lead to rapid opsonophagocytosis by phagocytic cells. Thus, antibody-mediated complement may have an effect via direct destruction or a pathogen clearance. CDC is a protective mechanism in mAbs against influenza virus,59,60 vaccinia virus,61 cytomegalovirus,62 and HIV.50,51 However, complement activation may contribute to disease severity in both dengue virus (DV)63,64 and HIV infection.65,66
first member of the cascade, C1q, to deposit on the surface of the pathogen.56 C1q, a pentamer of C1 molecules, can interact with IgM (also a pentamer) or multimerized IgG3 and IgG1 antibodies via their Fc-domains, once they have bound to the surface of a pathogen.57,58 C1q binding then leads to a cascade of enzymatic reactions and catalytic events, wherein additional members of the complement cascade deposit, forming the membrane attack complex (MAC) that ruptures the membrane of the pathogen and death. The deposition of complement components, midway in the formation of the MAC, such as C3b or C5b, can lead to rapid opsonophagocytosis by phagocytic cells. Thus, antibody-mediated complement may have an effect via direct destruction or a pathogen clearance. CDC is a protective mechanism in mAbs against influenza virus,59,60 vaccinia virus,61 cytomegalovirus,62 and HIV.50,51 However, complement activation may contribute to disease severity in both dengue virus (DV)63,64 and HIV infection.65,66
Antibody-mediated adaptive cell activation occurs when T cells and B cells, which both express complement receptors and FcRs,67,68 are modulated by the presence of antibody-pathogen complexes. For example, IC interactions with FcγRIIB on B cells temper B-cell activation, helping to control inflammatory immune responses.69,70 Complement receptors on T cells play a critical role in the initiation of the T-cell response, allowing ICs that may form immediately after infection to drive rapid activation and maturation of T cells.71 These processes are known as antibody-mediated immunomodulation and have important therapeutic implications.
Antibody-dependent enhancement (ADE) is the process by which antibodies either facilitate the uptake of pathogens into cells or contribute to the pathogenesis associated with the infection. ADE is principally mediated by IgG antibodies; it has also been shown to be mediated by IgM and complement or IgA antibodies.72, 73, 74 and 75 For example, in the setting of DV infection, preexisting non-neutralizing or sub-neutralizing concentrations of DV-specific antibodies have been shown to facilitate DV uptake upon reinfection into monocytes/macrophages, providing the virus with a direct conduit to their target cells thereby promoting infection and disease.76, 77 and 78 Specifically, cross-reactive antibodies from the first infection form ICs upon reinfection with a different serotype of DV; these stabilize the virus and promote viral uptake by macrophages, leading to dengue hemorrhagic fever and shock syndrome.79, 80, 81 and 82 ADE has been observed in other flaviviruses such as Zika virus, where in vitro studies show both convalescent plasmid therapy and mAbs enhance infection.83 In an animal model, SARS-1 pox viral vector–based vaccination of nonhuman primates induced antibodies that enhanced inflammation and pathology in the lungs, rather than enhance infection,84,85 pointing to a second mechanism by which antibodies could contribute to enhanced disease. Thus, understanding both the immune-protective and also potential pathologic activities of antibodies is key to harnessing their full vaccine and therapeutic potential.86
IMPORTANCE OF ANTIBODIES IN CONTROL OF COVID-19
Following SARS-CoV-2 infection, SARS-CoV-2-specific antibody development occurs in a predictable pattern (Figure 8.3). First, IgM antibodies appear rapidly within the first weeks of infection, followed closely by IgA antibodies in the mucosal membranes. Then, IgG antibodies appear, peaking at 3 to 7 weeks postsymptom onset.87 Neutralizing Abs, 90% of which target the RBD,88 appear within 7 to 15 days following symptom onset, increasing over time, then level off and decay,89 similar to natural decay patterns observed for nAbs to other common coronaviruses.90 This decay process renders previously infected individuals newly susceptible to infection every 2 to 4 years.91 SARS-CoV-2-specific antibodies primarily target the immunogenic S and nucleocapsid (N) proteins, particularly the highly immunogenic RBD.
![]() FIGURE 8.3 Profile of COVID-19 antibody response. Ig, immunoglobulin; PCR, polymerase chain reaction. |
Antibody production correlates with severity of disease, with the highest number of antibodies observed in individuals with severe COVID-19 and the lowest levels detected in asymptomatic cases.92,93 Thus, antibody levels are linked to the level of viral replication, or antigen levels, suggesting that individuals with more severe disease likely harbor higher levels of virus in their lungs, triggering stronger B-cell responses and thus eliciting higher levels of antibodies.94,95 Individuals with milder disease also show enhanced FcR binding, Fc-effector function, and neutralization, along with an increased number of antibody subclasses present.96, 97 and 98 Individuals with mild disease also have lower viral loads and lower antibodies. This suggests that qualitative differences in the antiviral functions of
antibodies, rather than quantitative levels, are likely key to control and clear infection. Dysfunctional Fc-effector functions have been linked to poor clinical outcome and have been associated with altered Fc-glycosylation.99,100 Specifically, decreased Fc fucosylation and increased titers of proinflammatory but poorly functional IgG2 antibodies are observed in severely ill COVID-19 patients, especially in male patients that typically suffer from enhanced morbidity and mortality in COVID-19.99 Collectively, these data suggest that in natural infection, Fc-effector functions may play a critical role in the control and clearance of infection, resulting in milder disease.
antibodies, rather than quantitative levels, are likely key to control and clear infection. Dysfunctional Fc-effector functions have been linked to poor clinical outcome and have been associated with altered Fc-glycosylation.99,100 Specifically, decreased Fc fucosylation and increased titers of proinflammatory but poorly functional IgG2 antibodies are observed in severely ill COVID-19 patients, especially in male patients that typically suffer from enhanced morbidity and mortality in COVID-19.99 Collectively, these data suggest that in natural infection, Fc-effector functions may play a critical role in the control and clearance of infection, resulting in milder disease.
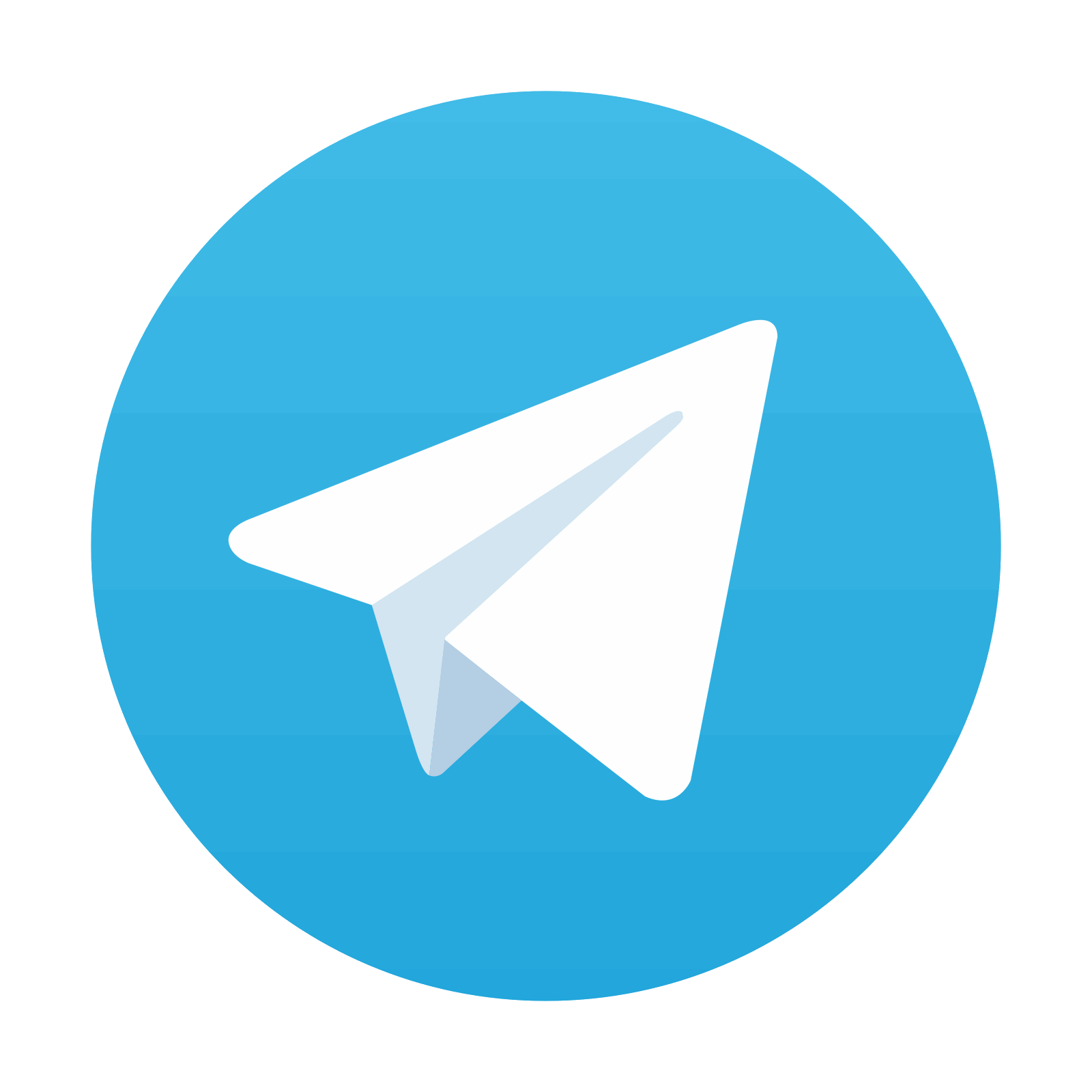
Stay updated, free articles. Join our Telegram channel

Full access? Get Clinical Tree
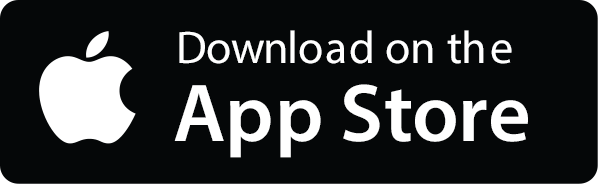
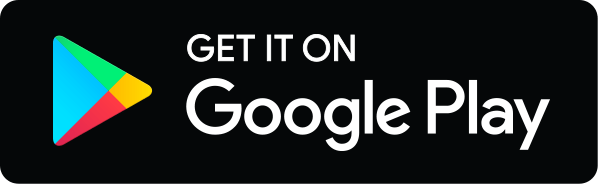