OBJECTIVES
After reading this chapter, youshould be able to:
Outline the processes involved in the secretion of H+ into the tubules and discuss the significance of these processes in the regulation of acid–base balance.
Define acidosis and alkalosis, and give (in mEq/L and pH) the normal mean andthe range of H+ concentrations in blood that are compatible with health.
List the principal buffers in blood, interstitial fluid, and intracellular fluid, and, using the Henderson-Hasselbalch equation, describe what is unique about the bicarbonate buffer system.
Describe the changes in blood chemistry that occur during the development of metabolic acidosis and metabolic alkalosis, and the respiratory and renal compensations for these conditions.
Describe the changes in blood chemistry that occur during the development of respiratory acidosis and respiratory alkalosis, and the renal compensation forthese conditions.
INTRODUCTION
The kidneys play a key role in the maintenance of acid–base balance and to do this they must excrete acid in the amount equivalent to the production of nonvolatile acids in the body. The production of nonvolatile acids will vary with diet, metabolism, and disease. The kidneys must also filter and reabsorb plasma bicarbonate, and thus prevent the loss of bicarbonate in the urine. Both processes are linked physiologically, due to the nephron’s ability to secrete H+ ions into the filtrate.
RENAL H+ SECRETION
The cells of the proximal and distal tubules, like the cells of the gastric glands (see Chapter 25), secrete hydrogen ions. Hydrogen secretion also occurs in the collecting ducts. The transporter that is responsible for H+ secretion in the proximal tubules is the Na–H exchanger (primarily NHE3) (Figure 39–1). This is an example of secondary active transport; Na+ is moved from the inside of the cell to the interstitium by Na, K ATPase on the basolateral membrane, which keeps intracellular Na+ low, thus establishing the drive for Na+ to enter the cell, via the Na–H exchanger, from the tubular lumen. The Na–H exchanger secretes H+ into the lumen in exchange for Na+.
The secreted H+ ion combines with filtered HCO3– to form H2CO3 and the presence of carbonic anhydrase on the apical membrane of the proximal tubule catalyzes the formation of H2O and CO2 from H2CO3. The apical membrane of epithelial cells lining the proximal tubule is permeable to CO2 and H2O, and they enter the tubule rapidly. Eighty percent of the filtered load of HCO3– is reabsorbed in the proximal tubule.
Inside the cell, carbonic anhydrase is also present and can catalyze the formation of H2CO3 from CO2 and H2O. H2CO3 dissociates into H+ ions and HCO3–; the H+ is secreted into the tubular lumen, as mentioned above, and the HCO3– that is formed diffuses into the interstitial fluid. Thus, for each H+ ion secreted, one Na+ ion and one HCO3– ion enter the interstitial fluid. Because carbonic anhydrase catalyzes the formation of H2CO3, drugs that inhibit carbonic anhydrase depress both secretion of acid by the proximal tubules and the reactions that depend on it.
Some evidence suggests that H+ is secreted in the proximal tubules by other types of transporters, but the evidence for these additional transporters is controversial, and in any case, their contribution is small relative to that of the Na–H exchanger mechanism.
This is in contrast to what occurs in the distal tubules and collecting ducts, where H+ secretion is relatively independent of Na+ in the tubular lumen. In this part of the tubule, most H+ is secreted by an ATP-driven proton pump. Aldosterone acts on this pump to increase distal H+ secretion. The I cells in this part of the renal tubule secrete acid and, like the parietal cells in the stomach, contain abundant carbonic anhydrase and numerous tubulovesicular structures. There is evidence that the H+-translocating ATPase that produces H+ secretion is located in these vesicles as well as in the apical cell membrane and that, in acidosis, the number of H+ pumps is increased by insertion of these tubulovesicles into the apical cell membrane. Some of the H+ is additionally secreted by H–K+ ATPase. The I cells also contain anion exchanger 1 (AE1, formerly known as Band 3), an anion exchange protein, in their basolateral cell membranes. This protein may function as a Cl/HCO3 exchanger for the transport of HCO3– to the interstitial fluid.
The amount of acid secreted depends on the subsequent events that modify the composition of the tubular urine. The maximal H+ gradient against which the transport mechanisms can secrete in humans corresponds to a urine pH of about 4.5; that is, an H+ concentration in the urine that is 1000 times the concentration in plasma. pH 4.5 is thus the limiting pH. This is normally reached in the collecting ducts. If there were no buffers that “tied up” H+ in the urine, this pH would be reached rapidly, and H+ secretion would stop. However, three important reactions in the tubular fluid remove free H+, permitting more acid to be secreted (Figure 39–2). These are the reactions of H+ with HCO3– to form CO2 and H2O (discussed above), with HPO42– to form H2PO4– (titratable acids), and with NH3 to form NH4+.
The three buffers of importance in the renal handling of acid and its secretion into the lumen are thus bicarbonate, dibasic phosphate, and ammonia. On an average diet, approximately 40% of nonvolatile acids (about 30 mEq/day), produced by the body in the course of various metabolic reactions is excreted as titratable acid (ie, phosphate system) and 60% of nonvolatile acid (about 50 mEq/day) is excreted as NH4+. The pK’ of the bicarbonate system is 6.1, that of the dibasic phosphate system is 6.8, and that of the ammonia system is 9.0. The concentration of HCO3– in the plasma, and consequently in the glomerular filtrate, is normally about 24 mEq/L, whereas that of phosphate is only 1.5 mEq/L. Therefore, in the proximal tubule, most of the secreted H+ reacts with HCO3– as described above, to form H2CO3 (Figure 39–2) and this enters the cell as CO2 and H2O following the action of carbonic anhydrase in the brush border of the proximal tubule cells. The CO2 entering the tubular cells adds to the pool of CO2 available to form H2CO3. Because most of the H+ is removed from the tubule, the pH of the fluid is changed very little. This is the mechanism by which HCO3– is reabsorbed; for each mole of HCO3– removed from the tubular fluid, 1 mol of HCO3– diffuses from the tubular cells into the blood, although it is important to note that it is not the same mole that disappeared from the tubular fluid. About 4500 mEq of HCO3– are filtered and reabsorbed each day.
Secreted H+ also reacts with dibasic phosphate (HPO 42–) to form monobasic phosphate (H2PO4–). This happens to the greatest extent in the distal tubules and collecting ducts, because it is here that the phosphate that escapes proximal reabsorption is greatly concentrated by the reabsorption of water. H+ ions are also known to combine to a minor degree with other buffer anions.
The ammonia buffering system allows secreted H+ to combine with NH3, and this occurs in the proximal tubule (where NH3 is made, see below) and in the distal tubules. The pK’ of the ammonia system is 9.0, and the ammonia system is titrated only from the pH of the urine to pH 7.4, so it contributes very little to the titratable acidity. Each H+ ion that reacts with the buffers contributes to the urinary titratable acidity, which is measured by determining the amount of alkali that must be added to the urine to return its pH to 7.4, the pH of the glomerular filtrate. However, the titratable acidity obviously measures only a fraction of the acid secreted, since it does not account for the H2CO3 that has been converted to H2O and CO2.
The reabsorption of HCO3– is crucial to the maintenance of acid–base balance, as a loss of a single HCO3– ion in the urine would be the equivalent of adding a H+ ion to the blood. However, the kidneys have the ability to replenish the body with new bicarbonate ions. This occurs when H+ ions are removed from the body as NH4+ or titratable acid, as there is formation of new bicarbonate within the cells, and this enters the blood (ie, these bicarbonate ions are not those originally filtered, and yet they still enter the blood).
As mentioned above, reactions in the renal tubular cells produce NH4+ and HCO3–. NH4+ is in equilibrium with NH3 and H+ in the cells. Because the pK’ of this reaction is 9.0, the ratio of NH3 to NH4+ at pH 7.0 is 1:100 (Figure 39–3). However, NH3 is lipid-soluble and diffuses across the cell membranes down its concentration gradient into the interstitial fluid and tubular urine. In the urine it reacts with H+ to form NH4+, and the NH4+ remains “trapped” in the urine.
The principal reaction producing NH4+ in cells is conversion of glutamine to glutamate. This reaction is catalyzed by the enzyme glutaminase, which is abundant in renal tubular cells (Figure 39–3). Glutamic dehydrogenase catalyzes the conversion of glutamate to α-ketoglutarate, with the production of more NH4+. Subsequent metabolism of α-ketoglutarate utilizes 2H+, freeing 2HCO3–.
In chronic acidosis, the amount of NH4+ excreted at any given urine pH also increases, because more NH3 enters the tubular urine. The effect of this adaptation of NH3 secretion, the cause of which is unsettled, is further removal of H+ from the tubular fluid and consequently a further enhancement of H+ secretion by the renal tubules and excretion in the urine. Because the amount of phosphate buffer filtered at the glomerulus cannot be increased, urinary excretion of acid via the phosphate buffer system is limited. The production of NH4+ by the renal tubules is the only way the kidneys can remove even the normal amount, much less an increased amount, of nonvolatile acid produced in the body.
In the inner medullary cells of the collecting duct, the main process by which NH3 is secreted into the urine and then changed to NH4+, is called nonionic diffusion (see Chapter 2), thereby maintaining the concentration gradient for diffusion of NH3. In the proximal tubule, nonionic diffusion of NH4+ is less important because NH4+ can be secreted into the lumen, often by replacing H+ on the Na–H exchanger.
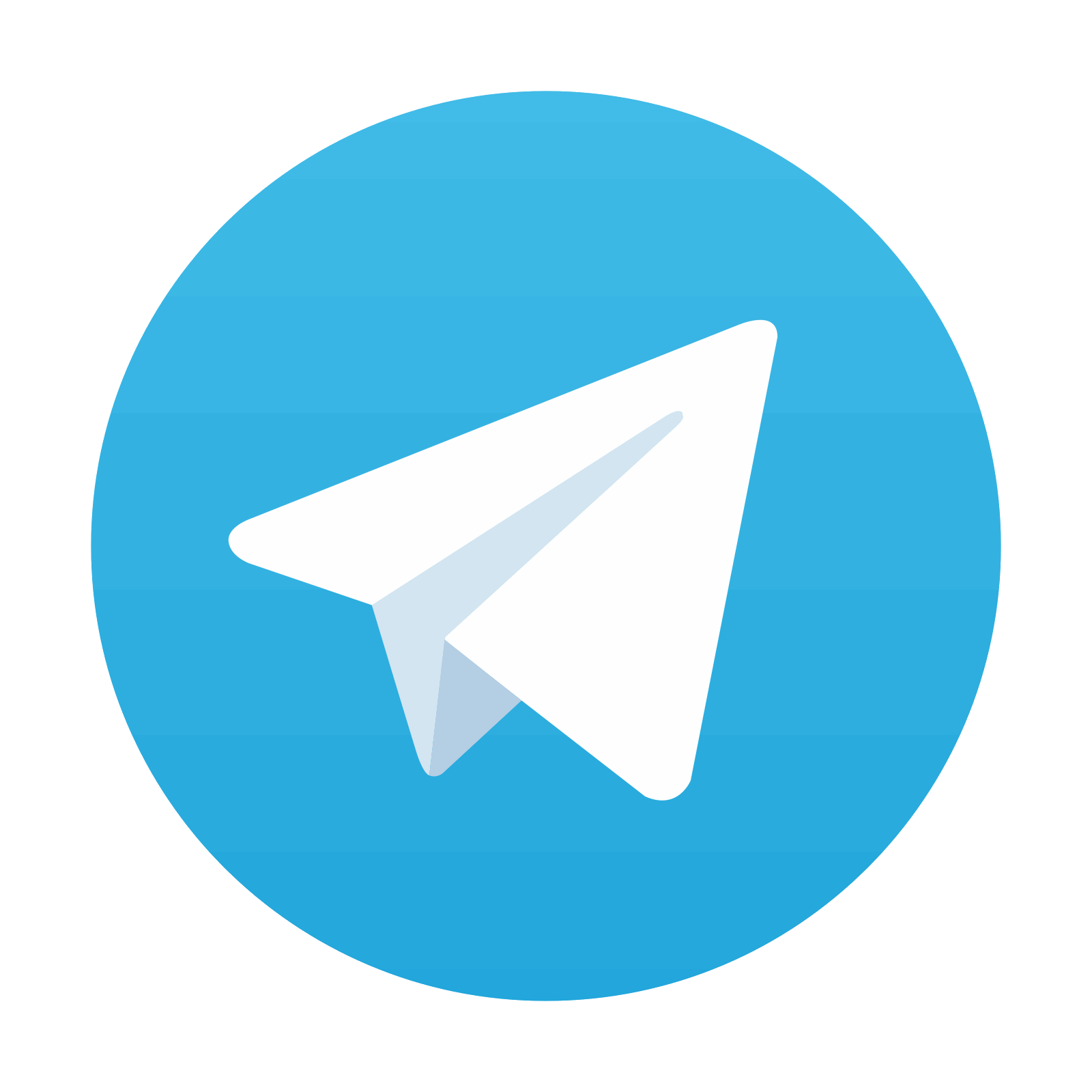
Stay updated, free articles. Join our Telegram channel

Full access? Get Clinical Tree
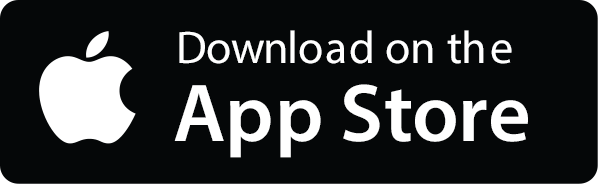
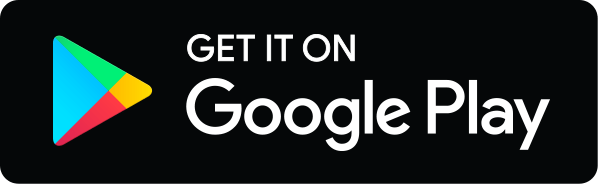